Learning Objectives
By the end of this section, you will be able to:
- Illustrate covalent bond formation with Lewis electron dot diagrams.
- Draw Lewis structures depicting the bonding in simple molecules
Let us illustrate a covalent bond by using H atoms, with the understanding that H atoms need only two electrons to fill the first shell. Each H atom starts with a single electron in its valence shell:The two H atoms can share their electrons:
We can use circles to show that each H atom has two electrons around the nucleus, completely filling each atom’s valence shell:
Because each H atom has a filled valence shell, this bond is stable, and we have made a diatomic hydrogen molecule. It is common to represent the covalent bond with a dash connecting the two elemental symbols, instead of with two dots:
H–H
Because two atoms are sharing one pair of electrons, this covalent bond is called a single bond.
As another example, consider fluorine. F atoms have seven electrons in their valence shell:These two atoms can do the same thing that the H atoms did; they share their unpaired electrons to make a covalent bond.
Note that each F atom has a complete octet around it now:
We can also write this using a dash to represent the shared electron pair:
There are two different types of electrons in the fluorine diatomic molecule. The bonding electron pair makes the covalent bond. Each F atom has three other pairs of electrons that do not participate in the bonding; they are called lone electron pairs, or nonbonding pairs. Each F atom has one bonding pair and three lone pairs of electrons.
Covalent bonds can be made between different elements as well. One example is HF. Each atom starts out with an odd number of electrons in its valence shell:The two atoms can share their unpaired electrons to make a covalent bond:
We note that the H atom has a full valence shell with two electrons, while the F atom also has a full shell, with eight electrons. This set of eight is referred to as an octet.
Example 1
Use Lewis electron dot diagrams to illustrate the covalent bond formation in HBr.
Solution
HBr is very similar to HF, except that it has Br instead of F. The atoms are as follows:The two atoms can share their unpaired electron:
More than two atoms can participate in covalent bonding, although any given covalent bond will be between two atoms only. Consider H and O atoms:The H and O atoms can share an electron to form a covalent bond:
The H atom has a complete valence shell. However, the O atom has only seven electrons around it, which is not a complete octet. We fix this by including a second H atom, whose single electron will make a second covalent bond with the O atom:
Now the O atom has a complete octet around it, and each H atom has two electrons, filling its valence shell. This is how a water molecule, H2O, is made.
Note that it does not matter on what side the second H atom is positioned. A perfectly legitimate structure could show the hydrogen atoms on opposite sides of the oxygen atom.
Example 2
Use a Lewis electron dot diagram to show the covalent bonding in NH3.
Solution
The N atom has the following Lewis electron dot diagram:It has three unpaired electrons, each of which can make a covalent bond by sharing electrons with an H atom. The electron dot diagram of NH3 is as follows:
The Octet Rule
The tendency of atoms of the main group elements (the tall columns on the periodic table) to form enough bonds to obtain eight valence electrons is known as the octet rule.
The number of bonds that an atom can form can often be predicted from the number of electrons needed to reach an octet (eight valence electrons); this is especially true of the nonmetals of the second period of the periodic table (C, N, O, and F). For example, each atom of a group 14 element has four electrons in its outermost shell and therefore requires four more electrons to reach an octet. These four electrons can be gained by forming four covalent bonds, as illustrated here for carbon in CCl4 (carbon tetrachloride) and silicon in SiH4 (silane). Because hydrogen only needs two electrons to fill its valence shell, it is an exception to the octet rule.
Group 15 elements such as nitrogen have five valence electrons in the atomic Lewis symbol: one lone pair and three unpaired electrons. To obtain an octet, these atoms form three covalent bonds, as in NH3 (ammonia). Oxygen and other atoms in group 16 obtain an octet by forming two covalent bonds:
Double and Triple Bonds
As previously mentioned, when a pair of atoms shares one pair of electrons, we call this a single bond. However, a pair of atoms may need to share more than one pair of electrons in order to achieve an octet. A double bond forms when two pairs of electrons are shared between a pair of atoms, as between the carbon and oxygen atoms in CH2O (formaldehyde) and between the two carbon atoms in C2H4 (ethylene):
A triple bond forms when three electron pairs are shared by a pair of atoms, as in carbon monoxide (CO) and the cyanide ion (CN–):
Writing Lewis Structures with the Octet Rule
For very simple molecules and molecular ions, we can write the Lewis structures by merely pairing up the unpaired electrons on the constituent atoms. See these examples:
For more complicated molecules and molecular ions, it is helpful to follow the step-by-step procedure outlined here:
- Determine the total number of valence (outer shell) electrons. For cations, subtract one electron for each positive charge. For anions, add one electron for each negative charge.
- Draw a skeleton structure of the molecule or ion, arranging the atoms around a central atom. (Generally, the least electronegative element should be placed in the center.) Connect each atom to the central atom with a single bond (one electron pair).
- Distribute the remaining electrons as lone pairs on the terminal atoms (except hydrogen), completing an octet around each atom.
- Place all remaining electrons on the central atom.
- Rearrange the electrons of the outer atoms to make multiple bonds with the central atom in order to obtain octets wherever possible.
Complications can arise even when using this sytematic approach. For the purposes of this introduction we do not need to consider many of them.
Let us determine the Lewis structures of SiH4, CHO2−, NO+, and OF2 as examples in following this procedure:
- Determine the total number of valence (outer shell) electrons in the molecule or ion.
- For a molecule, we add the number of valence electrons on each atom in the molecule:
[latex]\begin{array}{r r l} \text{SiH}_4 & & \\[1em] & \text{Si: 4 valence electrons/atom} \times 1 \;\text{atom} & = 4 \\[1em] \rule[-0.5ex]{21em}{0.1ex}\hspace{-21em} + & \text{H: 1 valence electron/atom} \times 4 \;\text{atoms} & = 4 \\[1em] & & = 8 \;\text{valence electrons} \end{array}[/latex]
- For a negative ion, such as CHO2−, we add the number of valence electrons on the atoms to the number of negative charges on the ion (one electron is gained for each single negative charge):
[latex]\begin{array}{r r l} {\text{CHO}_2}^{-} & & \\[1em] & \text{C: 4 valence electrons/atom} \times 1 \;\text{atom} & = 4 \\[1em] & \text{H: 1 valence electron/atom} \times 1 \;\text{atom} & = 1 \\[1em] & \text{O: 6 valence electrons/atom} \times 2 \;\text{atoms} & = 12 \\[1em] \rule[-0.5ex]{21.5em}{0.1ex}\hspace{-21.5em} + & 1\;\text{additional electron} & = 1 \\[1em] & & = 18 \;\text{valence electrons} \end{array}[/latex]
- For a positive ion, such as NO+, we add the number of valence electrons on the atoms in the ion and then subtract the number of positive charges on the ion (one electron is lost for each single positive charge) from the total number of valence electrons:
[latex]\begin{array}{r r l} \text{NO}^{+} & & \\[1em] & \text{N: 5 valence electrons/atom} \times 1 \;\text{atom} & = 5 \\[1em] & \text{O: 6 valence electrons/atom} \times 1 \;\text{atom} & = 6 \\[1em] \rule[-0.5ex]{21em}{0.1ex}\hspace{-21em} + & -1 \;\text{electron (positive charge)} & = -1 \\[1em] & & = 10 \;\text{valence electrons} \end{array}[/latex]
- Since OF2 is a neutral molecule, we simply add the number of valence electrons:
[latex]\begin{array}{r r l} \text{OF}_{2} & & \\[1em] & \text{O: 6 valence electrons/atom} \times 1 \;\text{atom} & = 6 \\[1em] \rule[-0.5ex]{21em}{0.1ex}\hspace{-21em} + & \text{F: 7 valence electrons/atom} \times 2 \;\text{atoms} & = 14 \\[1em] & & = 20 \;\text{valence electrons} \end{array}[/latex]
- For a molecule, we add the number of valence electrons on each atom in the molecule:
- Draw a skeleton structure of the molecule or ion, showing the connectivity between central atoms and starting with single bonds. Ions are given brackets around the structure, indicating the charge outside the brackets:
When several arrangements of atoms are possible, as for CHO2−, we must be instructed or use experimental evidence as a guide.
- Distribute the remaining electrons as lone pairs on the terminal atoms (except hydrogen) to complete their valence shells with an octet of electrons.
- There are no remaining electrons on SiH4, so it is unchanged:
- There are no remaining electrons on SiH4, so it is unchanged:
- Place all remaining electrons on the central atom.
- For SiH4, CHO2−, and NO+, there are no remaining electrons; we already placed all of the electrons determined in Step 1.
- For OF2, we had 16 electrons remaining in Step 3, and we placed 12, leaving 4 to be placed on the central atom:
- Rearrange the electrons of the outer atoms to make multiple bonds with the central atom in order to obtain octets wherever possible.
- SiH4: Si already has an octet, so nothing needs to be done.
- CHO2−: We have distributed the valence electrons as lone pairs on the oxygen atoms, but the carbon atom lacks an octet:
- NO+: For this ion, we added eight valence electrons, but neither atom has an octet. We cannot add any more electrons since we have already used the total that we found in Step 1, so we must move electrons to form a multiple bond:
This still does not produce an octet, so we must move another pair, forming a triple bond:
- In OF2, each atom has an octet as drawn, so nothing changes.
Polyatomic ions are bonded together with covalent bonds, as seen in the example of CHO2−. Because they are ions, however, they participate in ionic bonding with other ions. So both major types of bonding can occur in one compound.
Example 3
NASA’s Cassini-Huygens mission detected a large cloud of toxic hydrogen cyanide (HCN) on Titan, one of Saturn’s moons. Titan also contains ethane (H3CCH3), acetylene (HCCH), and ammonia (NH3). What are the Lewis structures of these molecules?
Solution
- Calculate the number of valence electrons.HCN: (1 × 1) + (4 × 1) + (5 × 1) = 10H3CCH3: (1 × 3) + (2 × 4) + (1 × 3) = 14HCCH: (1 × 1) + (2 × 4) + (1 × 1) = 10NH3: (5 × 1) + (3 × 1) = 8
- Draw a skeleton and connect the atoms with single bonds. Remember that H is never a central atom:
- Where needed, distribute electrons to the terminal atoms:
HCN: six electrons placed on NH3CCH3: no electrons remainHCCH: no terminal atoms capable of accepting electronsNH3: no terminal atoms capable of accepting electrons
- Where needed, place remaining electrons on the central atom:
HCN: no electrons remainH3CCH3: no electrons remainHCCH: four electrons placed on carbonNH3: two electrons placed on nitrogen
- Where needed, rearrange electrons to form multiple bonds in order to obtain an octet on each atom:HCN: form two more C–N bondsH3CCH3: all atoms have the correct number of electronsHCCH: form a triple bond between the two carbon atomsNH3: all atoms have the correct number of electrons
Example 4
What is the proper Lewis electron dot diagram for CO2?
Solution
The central atom is a C atom, with O atoms as surrounding atoms. We have a total of 4 + 6 + 6 = 16 valence electrons. Following the rules for Lewis electron dot diagrams for compounds gives usThe O atoms have complete octets around them, but the C atom has only four electrons around it. The way to solve this dilemma is to make a double bond between carbon and each O atom:
Fullerene Chemistry
Carbon soot has been known to man since prehistoric times, but it was not until fairly recently that the molecular structure of the main component of soot was discovered. In 1996, the Nobel Prize in Chemistry was awarded to Richard Smalley, Robert
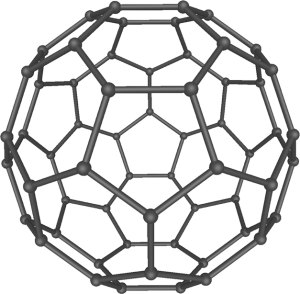
Curl, and Harold Kroto for their work in discovering a new form of carbon, the C60 buckminsterfullerene molecule. An entire class of compounds, including spheres and tubes of various shapes, were discovered based on C60. This type of molecule, called a fullerene, shows promise in a variety of applications. Because of their size and shape, fullerenes can encapsulate other molecules, atoms and ions, so they have shown potential in various applications from hydrogen storage to targeted drug delivery systems. They also possess unique electronic and optical properties that have been put to good use in solar powered devices and chemical sensors.
Food and Drink App: Vitamins and Minerals
Vitamins are nutrients that our bodies need in small amounts but cannot synthesize; therefore, they must be obtained from the diet. The word vitamin comes from “vital amine” because it was once thought that all these compounds had an amine group (NH2) in it. This is not actually true, but the name stuck anyway.
All vitamins are covalently bonded molecules. Most of them are commonly named with a letter, although all of them also have formal chemical names. Thus vitamin A is also called retinol, vitamin C is called ascorbic acid, and vitamin E is called tocopherol. There is no single vitamin B; there is a group of substances called the B complex vitamins that are all water soluble and participate in cell metabolism. If a diet is lacking in a vitamin, diseases such as scurvy or rickets develop. Luckily, all vitamins are available as supplements, so any dietary deficiency in a vitamin can be easily corrected.
A mineral is any chemical element other than carbon, hydrogen, oxygen, or nitrogen that is needed by the body. Minerals that the body needs in quantity include sodium, potassium, magnesium, calcium, phosphorus, sulfur, and chlorine. Essential minerals that the body needs in tiny quantities (so-called trace elements) include manganese, iron, cobalt, nickel, copper, zinc, molybdenum, selenium, and iodine. Minerals are also obtained from the diet. Interestingly, most minerals are consumed in ionic form, rather than as elements or from covalent molecules. Like vitamins, most minerals are available in pill form, so any deficiency can be compensated for by taking supplements.
Figure 2. Vitamin and Mineral Supplements
Every entry down through pantothenic acid is a vitamin, and everything from calcium and below is a mineral.
Key Concepts and Summary
Lewis dot structures are a useful system for modeling the architecture of molecules and polyatomic ions. Lone pairs and single, double, or triple bonds are used to indicate where the valence electrons are located around each atom in a Lewis structure. Most structures—especially those containing second row elements—obey the octet rule, in which every atom (except H) is surrounded by eight electrons.
Review-Reflect, Extend
Review-Reflect
4. Write Lewis structures for the following:
a) O2 b) H2CO c) AsF3 e) SiCl4
7. Methanol, H3COH, is used as the fuel in some race cars. Ethanol, C2H5OH, is used extensively as motor fuel in Brazil. Both methanol and ethanol produce CO2 and H2O when they burn. Write the chemical equations for these combustion reactions using Lewis structures instead of chemical formulas.
9. The arrangement of atoms in the amino acid serine is given here. Complete the Lewis structure of this molecule by adding multiple bonds and lone pairs. Do not add any more atoms.
18. Draw the Lewis electron dot diagram for each substance. Double or triple bonds may be needed.
a) CS2 b) NH2CONH2 (assume that the N and C atoms are the central atoms)
Extend
Lewis Structures are helpful because they are models that connect well with experimental data. They are symbolic representations that reveal structure on the microscopic level. But these models are only as good as their connection to macroscopic descriptions, based on observations of real substances.
One way to make experimental observations of bonds is to study the frequencies at which they resonate, similar to how vibrational tones on guitar strings are an indicator of how strong/thick the string is. Such studies reveal that carbon dioxide has only one kind of bond.
There is an alternative Lewis structure that can be drawn for CO2, different than the one in Example 4, that still follows the octet rule. Can you figure out what it is? Once you do, compare the strucutures of that one to the structure in Example 4, and use the information above to construct an explanation for which one is correct.
Answers to Review-Reflect
4. a)
In this case, the Lewis structure is inadequate to depict the fact that experimental studies have shown two unpaired electrons in each oxygen molecule.
b)
c)
d)
7.
9.
18.
Glossary
double bond: covalent bond in which two pairs of electrons are shared between two atoms
free radical: molecule that contains an odd number of electrons
hypervalent molecule: molecule containing at least one main group element that has more than eight electrons in its valence shell
Lewis structure: diagram showing lone pairs and bonding pairs of electrons in a molecule or an ion
Lewis symbol: symbol for an element or monatomic ion that uses a dot to represent each valence electron in the element or ion
lone pair: two (a pair of) valence electrons that are not used to form a covalent bond
octet rule: guideline that states main group atoms will form structures in which eight valence electrons interact with each nucleus, counting bonding electrons as interacting with both atoms connected by the bond
single bond: bond in which a single pair of electrons is shared between two atoms
triple bond: bond in which three pairs of electrons are shared between two atoms