Learning Goals
-
Describe how natural selection can result in adaptive evolution
-
Differentiate between stabilizing, directional and diversifying selection.
-
Describe how these different forces can lead to different outcomes in terms of the population variation
-
Explain how frequency-dependent selection can impact the genetic structure of a population
-
Describe the roles of sexual selection in the evolution of traits in populations
Introduction
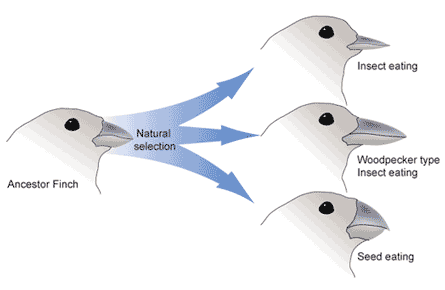
Natural selection only acts on the population’s heritable traits: selecting for beneficial alleles and thus increasing their frequency in the population, while selecting against deleterious alleles and thereby decreasing their frequency—a process known as adaptive evolution (Fig. 1). Natural selection acts on entire organisms though and not on individual alleles. Natural selection acts at the level of the individual; and involves differences in individual contributions to the gene pool of the next generation, known as an organism’s evolutionary (Darwinian) fitness. An individual may carry a very beneficial genotype with a resulting phenotype that, for example, increases the ability to reproduce (fecundity), but if that same individual also carries an allele that results in a fatal childhood disease, that fecundity phenotype will not be passed on to the next generation because the individual will not live to reach reproductive age.
Fitness is often quantifiable and is measured by scientists in the field. However, it is not the absolute fitness of an individual that counts, but rather how it compares to the other organisms in the population. This concept, called relative fitness, allows researchers to determine which individuals are contributing a differential number of offspring to the next generation, and thus, how the population might evolve. As natural selection influences the allele frequencies in a population, individuals can either become more or less genetically similar and as a result, the phenotypes displayed can also become less variable (more similar) or more variable (less similar).
Stabilizing Selection
Directional Selection
Review Question:
Diversifying Selection
Diversifying selection can also occur when environmental changes favor individuals on either end of the phenotypic spectrum (Fig. 4). Imagine a population of mice living at the beach where there is light-colored sand interspersed with patches of tall grass. In this scenario, light-colored mice that blend in with the sand would be favored, as well as dark-colored mice that can hide in the grass. Medium-colored mice, on the other hand, would not blend in with either the grass or the sand, and would thus be more likely to be eaten by predators. The result of this type of selection is increased genetic variance as the population becomes more diverse.
Review Question:
A) still 2 cm
Frequency-dependent Selection
In this scenario, orange males will be favored by natural selection when the population is dominated by blue males, blue males will thrive when the population is mostly yellow males, and yellow males will be selected for when orange males are the most populous. As a result, populations of side-blotched lizards cycle in the distribution of these phenotypes—in one generation, orange might be predominant, and then yellow males will begin to rise in frequency. Once yellow males make up a majority of the population, blue males will be selected. Finally, when blue males become common, orange males will once again be favored. Negative frequency-dependent selection serves to increase the population’s genetic variance by selecting for rare phenotypes, whereas positive frequency-dependent selection usually decreases genetic variance by selecting for common phenotypes.
Sexual Selection
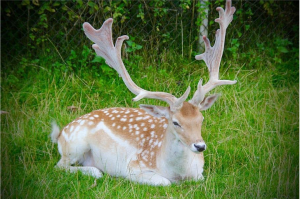
Males and females of certain species are often quite different from one another in ways beyond the reproductive organs. Males are often larger, for example, and display many elaborate colors and adornments, like the peacock’s tail, while females tend to be smaller and duller in decoration. Such differences are known as sexual dimorphisms, which arise from the fact that in many populations, particularly animal populations, there is more variance in the reproductive success of the males than there is of the females. That is, some males—often the bigger, stronger, or more decorated males—get the vast majority of the total mating, while others receive none.
This can occur because the males are better at fighting other males, or because females will choose to mate with the bigger or more decorated males (Fig. 6). In either case, this variation in reproductive success generates a strong selection pressure among males to successfully mate, resulting in the evolution of bigger body size and elaborate ornaments to get the females’ attention. Females, on the other hand, tend to mate less frequently; therefore, they are more likely to select more desirable males each time.
Sexual dimorphism varies widely among species, of course, and some species are even sex-role reversed. In such cases, females tend to have a greater variance in their reproductive success than males and are correspondingly selected for the bigger body size and elaborate traits usually characteristic of males (Fig. 7).
The good genes hypothesis states that males develop these impressive ornaments to show off their efficient metabolism or their ability to fight disease. Females then choose males with the most impressive traits because it signals their genetic superiority, which they will then pass on to their offspring. Though it might be argued that females should not be picky because it will likely reduce their number of offspring, if better males father more fit offspring, it may be beneficial. Fewer, healthier offspring may increase the chances of survival more than many, weaker offspring. In both the handicap principle and the good genes hypothesis, the trait is said to be an honest signal of the males’ quality, thus giving females a way to find the fittest mates— males that will pass the best genes to their offspring.
No Perfect Organism
Furthermore, natural selection can be constrained by the relationships between different polymorphisms. One morph may confer a higher fitness than another, but may not increase in frequency due to the fact that going from the less beneficial to the more beneficial trait would require intermediate morphs that are a less beneficial phenotype. Think back to the mice that live at the beach. Some are light-colored and blend in with the sand, while others are dark and blend in with the patches of grass. The dark-colored mice may be, overall, more fit than the light-colored mice, and at first glance, one might expect that through selection the gradual change to dark coloration would be favored. However, remember that the intermediate phenotype, a medium-colored coat, is not favorable for the mice—they cannot blend in with either the sand or the grass and are more likely to be eaten by predators. As a result, the gradual change to a dark coloration would not occur because those individuals that began displaying an intermediate phenotype would be less fit than those individuals who retained the light coloration.
Finally, it is important to understand that not all evolution is adaptive. While natural selection selects the fittest individuals and often results in a more fit population overall, other forces of evolution, including genetic drift and gene flow, often do the opposite: introducing deleterious alleles to the population’s gene pool. Evolution has no purpose—it is not changing a population into a preconceived ideal. It is simply the sum of the various forces described in this reading and how they influence the genetic and phenotypic variance of a population.
Summary
Because natural selection acts to increase the frequency of beneficial alleles and traits in a given environment while decreasing the frequency of deleterious qualities, it is adaptive evolution. Natural selection acts at the level of the individual, selecting for those that have a higher overall fitness compared to the rest of the population and resulting in differential reproductive success. If the fit phenotypes are those that are similar, natural selection will result in stabilizing selection, and an overall decrease in the population’s variation in those phenotypes will occur. Directional selection works to shift a population’s variance toward a new, fit phenotype, as environmental conditions change. In contrast, diversifying selection results in increased genetic variance by selecting for two or more distinct phenotypes.
Other types of selection include frequency-dependent selection, in which individuals with either common (positive frequency-dependent selection) or rare (negative frequency-dependent selection) are selected for. Finally, sexual selection results from the fact that one sex has more variance in the reproductive success than the other. As a result, males and females experience different selective pressures, which can often lead to the evolution of phenotypic differences, or sexual dimorphisms, between the two.
End of Section Review Questions:
Review: Sexual Selection 1
2) When males and females of a population look or act differently, it is referred to as?
Review: Sexual Selection 2
3) The good genes hypothesis is a theory that explains what?
CRITICAL THINKING QUESTION
Attributions
Footnotes
- [1]Sahar S. Hanania, Dhia S. Hassawi, and Nidal M. Irshaid, ftAllele Frequency and Molecular Genotypes of ABO Blood Group System in a Jordanian Population,ft Journal of Medical Sciences 7 (2007): 51-58, doi:10.3923/jms.2007.51.58.
- [2]A. J. Tipping et al., ftMolecular and Genealogical Evidence for a Founder Efect in Fanconi Anemia Families of the Afrikaner Population of South Africa,ft PNAS98, no. 10 (2001): 5734-5739, doi: 10.1073/pnas.091402398.
AttributionsText: Adapted from OpenStax Biology 2nd Edition, Biology 2e. OpenStax CNX. Nov 26, 2018 http://cnx.org/contents/8d50a0af-948b-4204-a71d-4826cba765b8@15.1.
Figure 1. Image courtesy of National Human Genome Research Institute’s Talking Glossary [Public domain], via Wikimedia Commons
Figure 2. Image modified from OpenStax Biology 2nd Edition, Biology 2e. OpenStax CNX. Nov 26, 2018 http://cnx.org/contents/8d50a0af-948b-4204-a71d-4826cba765b8@15.1
Figure 3. Modified by D. Jennings from image created by Pearson Scott Foresman [Public domain], via Wikimedia Commons
Figure 4. Image modified from OpenStax Biology 2nd Edition, Biology 2e. OpenStax CNX. Nov 26, 2018 http://cnx.org/contents/8d50a0af-948b-4204-a71d-4826cba765b8@15.1
Figure 5. Blue male image and orange male images from the public domain. Combined by D. Jennings
Figure 6. Male deer CC By 0, public domain
Figure 7. Spider: modification of work by ftSanba38ft/Wikimedia Commons; Duck: modification of work by Kevin Cole
D
C
A
D