Learning Goals
By the end of this reading you should be able to:
- Describe the similarities and differences in bacterial and archaeal structures
- Explain differences between the plasma membranes of archaea and bacteria
- Differentiate between gram-positive and gram-negative bacteria
- Describe the types of cell walls and cell wall components that exist in Archaea
- Identify the additional structures that are found in some Bacteria and Archaea
Introduction
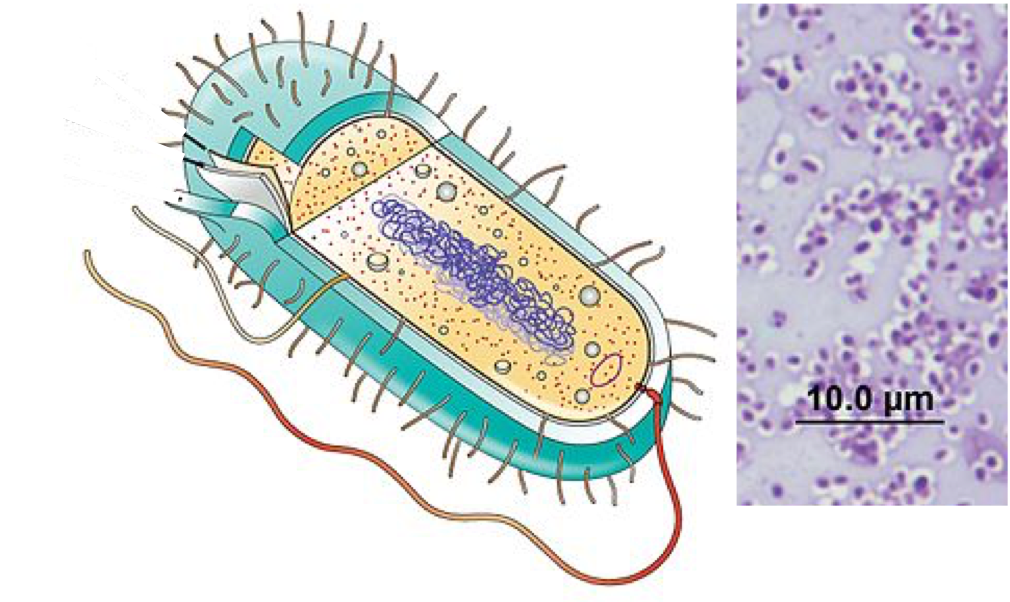
Early Earth had a very different atmosphere (contained less molecular oxygen) than it does today and was subjected to strong radiation; thus, the first organisms would have flourished where they were more protected, such as in ocean depths or beneath the surface of the Earth. At this time too, strong volcanic activity was common on Earth, so it is likely that these first organisms—the first prokaryotes—were adapted to very high temperatures. Early Earth was prone to geological upheaval and volcanic eruption and was subject to bombardment by mutagenic radiation from the sun. The first organisms were cells that could withstand these relatively harsh conditions.
These first cells and all the cells that evolved from them share four common components: 1) a plasma membrane, an outer covering that separates the cell’s interior from its surrounding environment; 2) cytoplasm, consisting of a jelly-like cytosol within the cell in which other cellular components are found; 3) DNA, the genetic material of the cell; and 4) ribosomes, which synthesize proteins. Additional structures are the result of evolutionary processes.
Basic Prokaryotic Structure
Prokaryotes are unicellular (single-celled) organisms that lack any internal membrane-bound structures, thus they lack a nucleus. In general that have a single circular chromosome located in an area of the cell referred to as the nucleoid or nucleoid region, which also contains some proteins and RNA that are associated with replication, transcription, translation, and DNA packaging. Most prokaryotes have a cell wall external to the plasma membrane. The components of this wall are different in the Archaea and Bacteria, which is one way in which these groups differ. This cell wall acts as an extra layer of protection, helps the cell maintain its shape, and prevents cell lysis.
Plasma membranes
The plasma or cell membrane is the boundary that defines the cell and controls the interactions that the cell has with its environment. Membranes exclude some molecules, allow some molecules to pass freely into or out of the cell, and can be involved in transmitting signals from outside to inside the cell. Because the membrane is the main way the cell interacts with the environment it must be both flexible and responsive to changes.
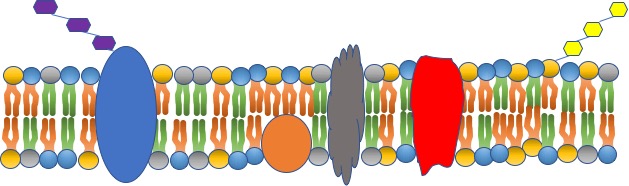
The integral proteins and lipids exist in the membrane as separate but loosely attached molecules. These resemble the separate, multicolored tiles of a mosaic picture, and they float, moving somewhat with respect to one another. The membrane is not like a balloon, however, that can expand and contract; rather, it is fairly rigid and can burst if penetrated or if a cell takes in too much water. However, because of its mosaic nature, a very fine needle can easily penetrate a plasma membrane without causing it to burst, and the membrane will flow and self-seal when the needle is extracted. In addition to the mosaic of proteins, lipids, and in some cases sterols and carbohydrates, the structure of the fatty acid chains of each phospholipid can also contribute to membrane properties. Saturated fatty acids help to provide structure while unsaturated tails can provide fluidity.
Review Question:
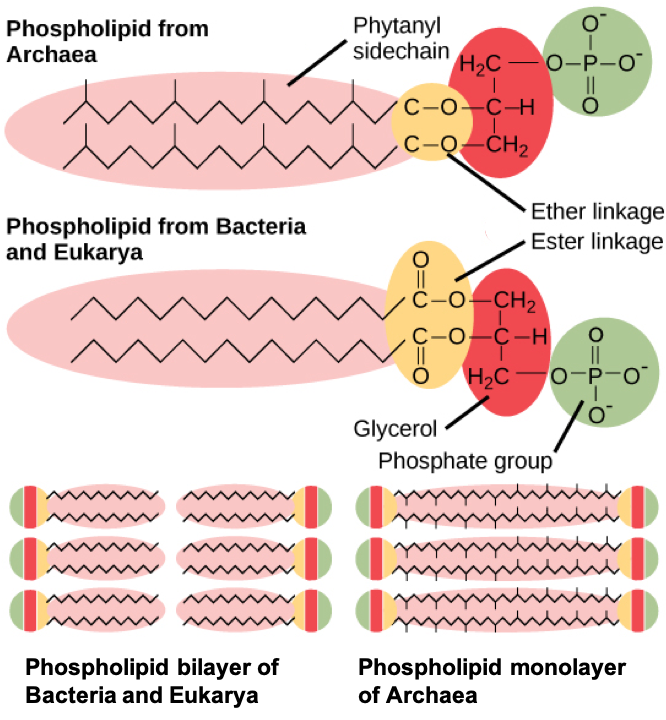
The membranes of Archaea contain unique molecules that are not found in the membranes of either bacterial or eukaryotic cells. Rather than the fatty acid chains linked to glycerol that are the key components of bacterial and eukaryotic cells, the archaeal membranes are composed of isoprene (phytanyl) chains linked to glycerol. These molecules allow some archaeal membranes to form lipid monolayers instead of bilayers. This formation provides additional structure to the membrane and can be beneficial in some of the extreme environments in which Archaea are found to live.
Thinking Question:
How might the structure of the Archaeal membrane relate to the ability of this group to adapt to extreme environmental conditions (heat, cold, etc)? Be sure to explain your reasoning.
The Cell Wall
The cell wall is a protective layer that surrounds some cells and gives them shape and rigidity. It is located outside the cell membrane and prevents osmotic lysis (bursting due to increasing volume). The chemical composition of the cell walls varies between archaea and bacteria, and also varies between bacterial species. Most prokaryotic cells live in a liquid environment that is often hypotonic to the cell. In addition, the cytoplasm of prokaryotic cells often has a high concentration of dissolved solutes making it hypertonic to the surrounding environment. Therefore, the osmotic pressure within the cell is relatively high, making a cell wall an essential component for survival. In addition, the cell wall is important in keeping out certain molecules, such as toxins, and in certain bacteria, the cell wall can contribute to the pathogenicity or disease–causing ability of the cell.
Review Question:
Bacterial cell walls contain peptidoglycan, a compound that is not found anywhere else, and additional ingredient molecules, making the bacterial cell wall a complex structure overall. Peptidoglycans are composed of alternating long polysaccharide chains of two glucose derivatives, N-acetylglucosamine (NAG) and N-acetylmuramic acid (NAM). A crosslink between the chains is formed by a tetrapeptide that extends off the NAM sugar unit and contains both L- and D-amino acids including D-glutamic acid and D-alanine. Proteins normally have only L-amino acids; as a consequence, many of our antibiotics work by mimicking D-amino acids and therefore have specific effects on bacterial cell wall development. There are more than 100 different forms of peptidoglycan.
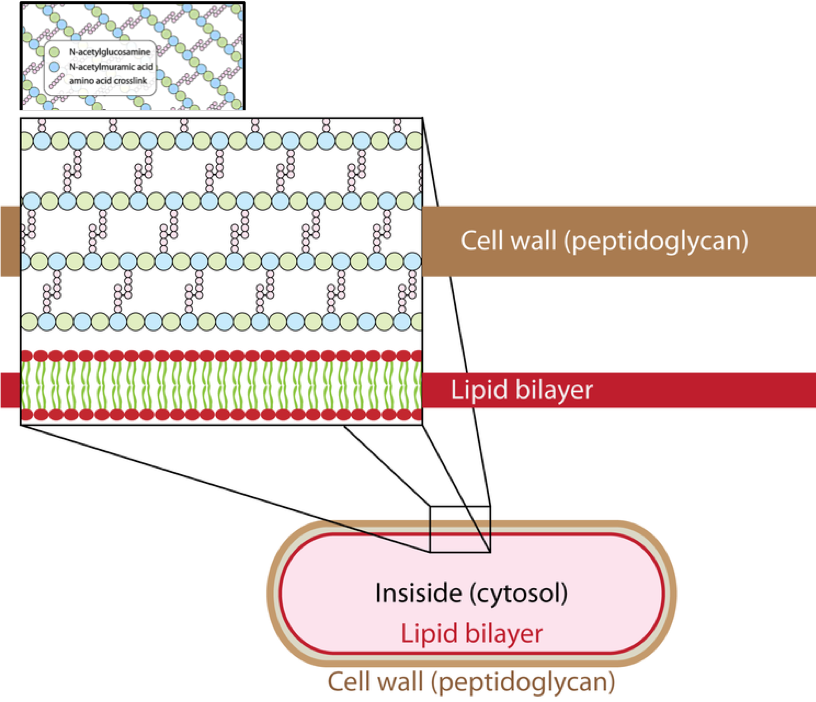
90% of bacteria that do have a cell wall typically have one of two types: Gram-positive or Gram-negative. They can be differentiated in the lab using a staining method named after its inventor, Danish scientist Hans Christian Gram (1853–1938). The different bacterial responses to the staining procedure are ultimately due to cell wall structure. Gram-positive organisms typically lack the outer membrane found in Gram-negative organisms. Up to 90 percent of the cell wall in Gram-positive bacteria is composed of peptidoglycan, and most of the rest is composed of acidic substances called teichoic acids. Teichoic acids may be covalently linked to lipids in the plasma membrane to form lipoteichoic acids. Lipoteichoic acids anchor the cell wall to the cell membrane.
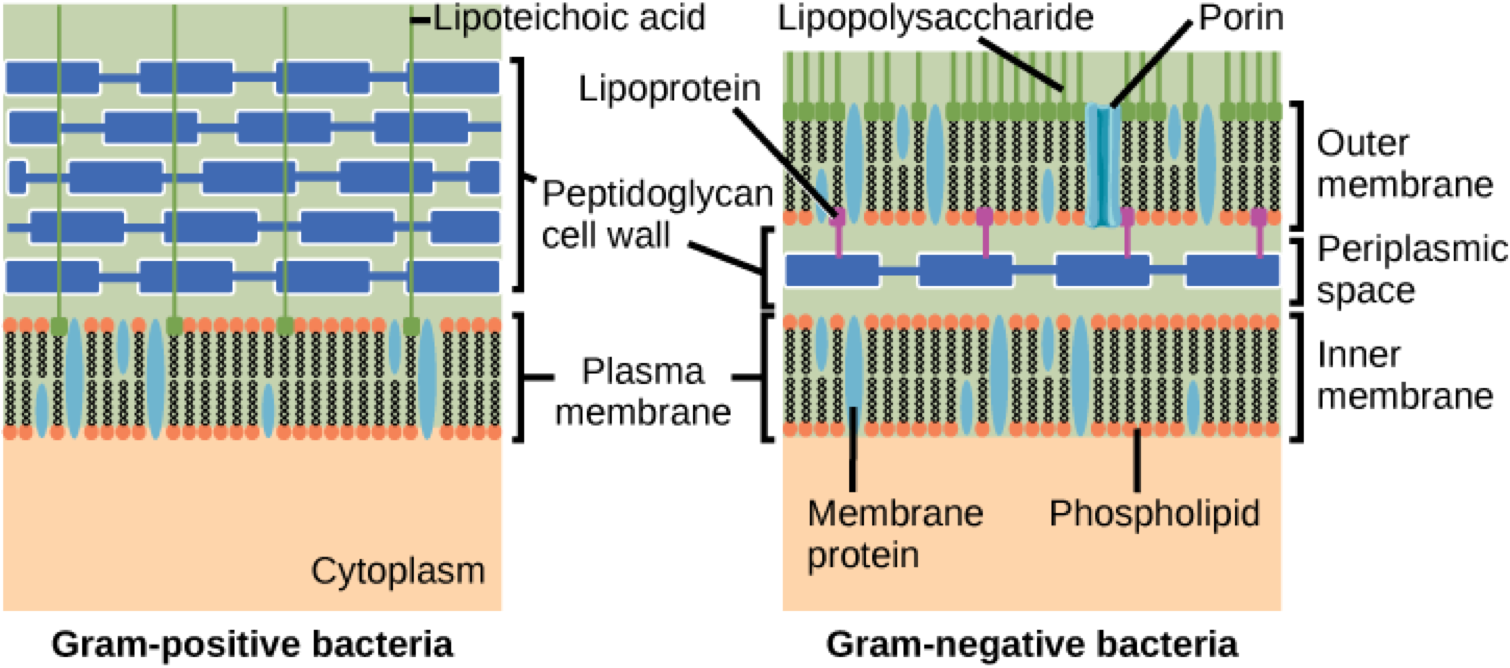
Gram-negative bacteria have a relatively thin cell wall composed of a few layers of peptidoglycan (only 10 percent of the total cell wall), surrounded by an outer envelope containing lipopolysaccharides (LPS) and lipoproteins. Porin proteins in this cell membrane allow substances to pass through the outer membrane of Gram-negative bacteria. This outer envelope is sometimes referred to as a second lipid bilayer and it is made up of three different components. The outermost part is composed of O-antigen or O-polysaccharide, beneath this is the core polysaccharide, and finally lipid A anchors the whole structure to the outer membrane of the cell.
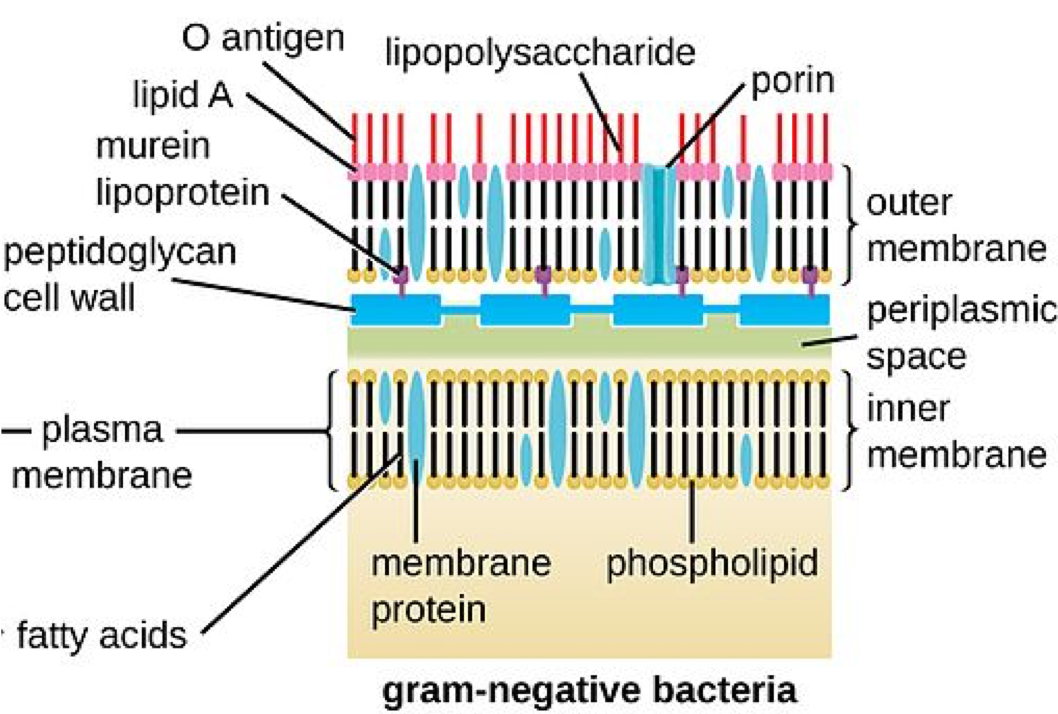
The LPS contributes to the net negative charge for the cell, helps to stabilize the outer membrane, and can provide protection from certain chemical substances by physically blocking access to parts of the cell wall. During infection of a host organism, the O-antigen in the LPS can trigger an immune response in an infected host, causing the generation of antibodies specific to that part of LPS. In addition, Lipid A can act as a toxin, specifically an endotoxin, causing general symptoms of illness such as fever and diarrhea. A large amount of lipid A released into the bloodstream can trigger endotoxic shock, a body-wide inflammatory response that can be life-threatening.
Review Question:
The cell walls of Archaea, unlike those of bacteria, do not contain peptidoglycan. In fact, archaea display a wide variety of cell wall types, each of which is adapted for the environment in which they are found. There are some archaea that lack a cell wall altogether.
A large number of Archaea have a proteinaceous S-layer made of either protein or glycoprotein, often anchored into the plasma membrane of the cell. This S-layer is considered to be part of the cell wall itself, unlike in Bacteria, where an S-layer is a structure in addition to the cell wall. For some Archaea, the S-layer is the only cell wall component, while in others it is joined by additional ingredients.
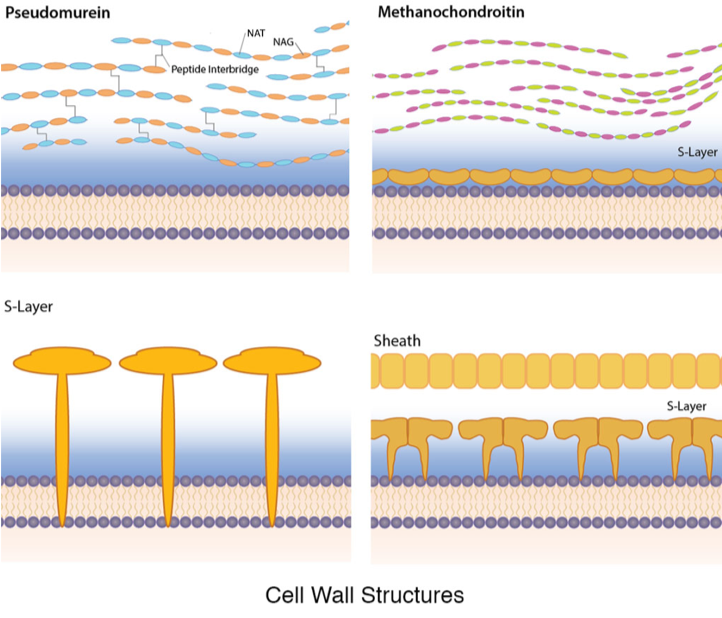
Some archaea contain a substance with a similar chemical structure to peptidoglycan, known as pseudomurein. Instead of N-acetylmuramic acid (NAM), it contains N-acetylalosaminuronic acid (NAT) linked to N-acetylglucosamine (NAG), with peptide interbridges to increase strength. Other archaeal cell walls contain the polymer methanochondroitin which is similar in composition to the connective tissue component chondroitin, found in vertebrates. Finally, there are archaea that have a protein sheath composed of a lattice structure similar to an S-layer. These cells are often found in filamentous chains, however, and the protein sheath encloses the entire chain, as opposed to individual cells.
Ribosomes
While archaea have ribosomes that are 70S in size, the same as bacteria, it was the rRNA nucleotide differences that provided scientists with the conclusive evidence to argue that archaea deserved a domain separate from the bacteria. In addition, archaeal ribosomes have a different shape than bacterial ribosomes, with proteins that are unique to archaea. This provides them with resistance to antibiotics that inhibit ribosomal function in bacteria.
Additional Structures
Many of the structures found in bacteria have been discovered in archaea as well, although sometimes it is obvious that each structure was evolved independently based on differences in substance and construction.
Cannulae: a structure unique to some marine Archaeal strains. These hollow tube-like structures appear to connect cells after division, eventually leading to a dense network composed of numerous cells and tubes. This could serve as a means of anchoring a community of cells to a surface.
Hamus: another structure unique to Archaea. This structure is a long helical tube with three hooks at the far end. Hami appears to allow cells to attach both to one another and to surfaces, encouraging the formation of a community.
Pilus (pl. pili): found in both Bacteria and Archaeal species. In Archaea, pili are composed of proteins most likely modified from the bacterial pilin. These tube-like structures have been shown to be used for attachment to surfaces.
Flagellum: Found in some species of Bacteria as well as some species of Archaea. The archaeal flagellum, while used for motility, differs so markedly from the bacterial flagellum that it has been proposed to call it an “archaellum,” to differentiate it from its bacterial counterpart. In both groups, the flagellum is used for movement, where the cell is propelled by the rotation of a rigid filament extending from the cell. After that the similarities end;
- The rotation of an archaeal flagellum is powered by ATP, as opposed to the proton motive force used in bacteria
- The proteins making up the archaeal flagellum are similar to the proteins found in bacterial pili, rather than the bacterial flagellum
- The archaeal flagellum filament is not hollow so growth occurs when flagellin proteins are inserted into the base of the filament, rather than being added to the end
- The filament is made up of several different types of flagellin, while just one type is used for the bacterial flagellum filament.
- Clockwise rotation pushes archaeal cells forward, while counterclockwise rotation pulls an archaeal cell backward. An alternation of runs and tumbles such as is seen in bacteria is not observed in archaea.
Summary
Prokaryotic cells and eukaryotic cells all have cell membranes, ribosomes, genetic material, and a cytosol. The main differentiation between them is the presence or absence of internal membrane-bound structures. Even within the prokaryotes, there are key differences in the structures of Archaeal and Bacterial cells. The membrane components vary, which results in membranes that have different properties and can be related to the environments in which these organisms live. In addition, there are key differences in the components and structures of the cell walls both within bacterial and archaeal groups as well as between the Bacteria and Archaea. Each component and structure can be related to the evolution of these organisms in different environments.
End of Section Review Questions:
4) How might the different structures found in Archaea and Bacteria have evolved? You should think about the mechanisms and patterns of evolution.
Extra: Review of Membrane Properties
In their saturated form, the fatty acids in phospholipid tails are saturated with bound hydrogen atoms. There are no double bonds between adjacent carbon atoms. This results in tails that are relatively straight. In contrast, unsaturated fatty acids do not contain a maximal number of hydrogen atoms, but they do contain some double bonds between adjacent carbon atoms; a double bond results in a bend in the string of carbons of approximately 30 degrees.
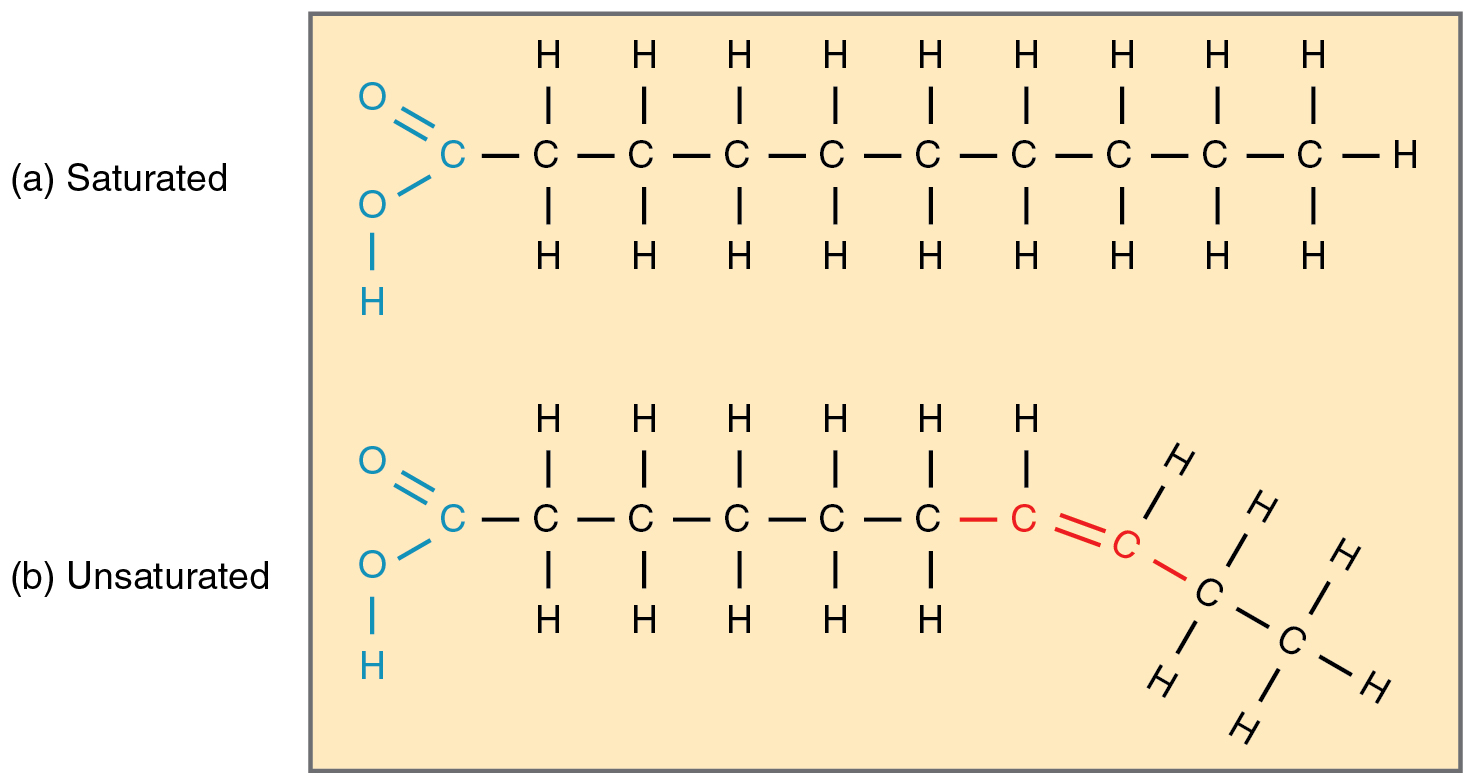
The other molecule that can play a role in membrane fluidity is steroids. In human cell membranes, the sterol that is most common is cholesterol. Like phospholipids, cholesterol is an amphipathic molecule. While the nonpolar portion of cholesterol tucks into the fatty acid part of the membrane, cholesterol’s hydroxyl (OH) group is hydrophilic and thus associates with the phosphate heads of the phospholipids. Part of the steroid rings of cholesterol is closely attracted to the phospholipid tails and help to immobilize the outer surface of the membrane, keeping it more rigid. However, when found in high concentrations, like the 20-50% proportion found in human cells, it can also help prevent the saturated fatty acid tails from condensing too much and thus aid in maintaining the fluidity of the cell.
References
OpenStax, Biology. OpenStax CNX. Nov 7, 2018 http://cnx.org/contents/185cbf87-c72e-48f5-b51e-f14f21b5eabd@11.6. 22.2 Structure of Prokaryotes
Microbiology. Linda Bruslind http://library.open.oregonstate.edu/microbiology/
Image Attribution
Figure 1.Image courtesy of CNX OpenStax [CC BY 4.0 (https://creativecommons.org/licenses/by/4.0)], via Wikimedia Commons (modified by D. Jennings)
Figure 2. Image created and provided by D. Jennings
Figure 3. Image courtesy of CNX OpenStax [CC BY 4.0 (https://creativecommons.org/licenses/by/4.0)], via Wikimedia Commons
Figure 4. Images courtesy of Bradleyhintze [CC0], from Wikimedia Commons (combined by D. Jennings)
Figure 5. Image courtesy of CNX OpenStax [CC BY 4.0 (https://creativecommons.org/licenses/by/4.0)], via Wikimedia Commons
Figure 6. Image courtesy of CNX OpenStax [CC BY 4.0 (https://creativecommons.org/licenses/by/4.0)], via Wikimedia Commons (modified by D. Jennings)
Figure 7. Image courtesy of Linda Bruslind Creative Commons Attribution-NonCommercial-ShareAlike 4.0 International License
Figure 8. Image courtesy of OpenStax College [CC BY 3.0 (https://creativecommons.org/licenses/by/3.0)], via Wikimedia Commons
B, C
B, D
A part of the LPS of some bacteria that can cause illness in another organism
C
a structure in living tissue which closely envelops another and is composed of protein
Energy that is generated by the transfer of protons or electrons across an energy-transducing membrane and that can be used for chemical, osmotic, or mechanical work.
B
B, C, E
A, B, D