Learning Goals
By the end of this reading you should be able to:
- List and describe the key characteristics of Eukaryotes
- Correlate energy production in prokaryotes to the changing conditions on early Earth
- Explain the evidence that supports the theory of endosymbiosis
- Describe the evidence to support the second endosymbiosis of plastids
Introduction
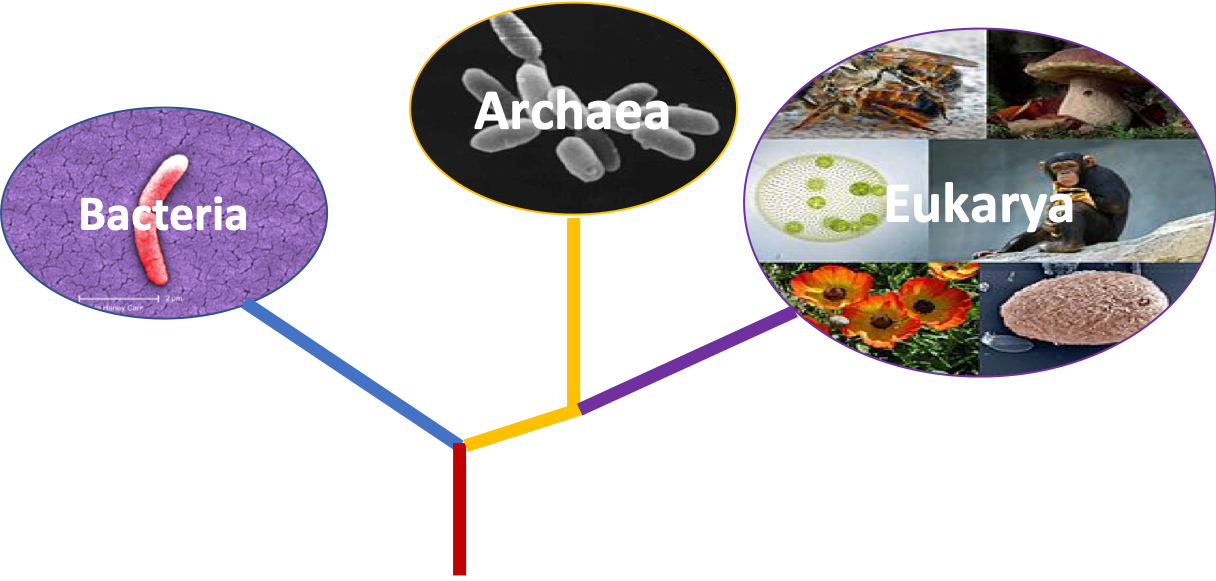
Living things fall into three large groups: Archaea, Bacteria, and Eukarya. The first two have prokaryotic cells, and the third contains all eukaryotes. A relatively sparse fossil record is available to help discern what the first members of each of these lineages looked like, so it is possible that all the events that led to the last common ancestor of extant eukaryotes will remain unknown. However, comparative biology of extant organisms and the limited fossil record provide some insight into the history of Eukarya. Data from these fossils have led comparative biologists to the conclusion that living eukaryotes are all descendants of a single common ancestor.
Characteristics of Eukaryotes
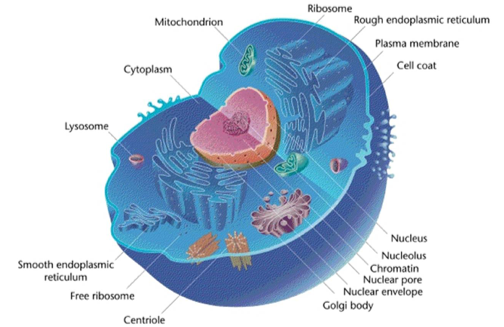
Mapping the characteristics found in all major groups of eukaryotes reveals that the following characteristics must have been present in the last common ancestor because these characteristics are present in at least some of the members of each major lineage. Some characteristics have been lost in some lineages over time.
- Cells with nuclei surrounded by a nuclear envelope with nuclear pores.
- Mitochondria. Some extant eukaryotes have very reduced remnants of mitochondria in their cells, whereas other members of their lineages have “typical” mitochondria.
- A cytoskeleton containing the structural and motility components called actin microfilaments and microtubules.
- Flagella and cilia, organelles associated with cell motility. Some extant eukaryotes lack flagella and/or cilia, but they are descended from ancestors that possessed them.
- Chromosomes, each consisting of a linear DNA molecule coiled around basic (alkaline) proteins called histones. The few eukaryotes with chromosomes lacking histones clearly evolved from ancestors that had them.
- Mitosis, a process of nuclear division wherein replicated chromosomes are divided and separated using elements of the cytoskeleton. Mitosis is universally present in eukaryotes.
- Sex, a process of genetic recombination unique to eukaryotes in which diploid nuclei at one stage of the life cycle undergo meiosis to yield haploid nuclei and a subsequent stage where two haploid nuclei fuse together to create a diploid zygote nucleus.
The Endosymbiotic Theory
Eukaryotic cells are differentiated from prokaryotic cells by a variety of features, one of which is the presence of internal membrane-bound structures (ex: nucleus, mitochondria, chloroplasts). How did these internal structures evolve? Before we can delve into that we need to talk about energy because it is one of the driving forces of life and there are some key points related to energy metabolism in prokaryotes and eukaryotes that we need to understand.
Prokaryotic Metabolism
While today’s atmosphere is about one-fifth of molecular oxygen (O2), geological evidence shows that it originally lacked O2. Without oxygen, aerobic respiration would not be expected, and living things would have relied on fermentation instead. About 3.5 billion years ago some prokaryotes began using energy from sunlight to power anabolic processes that reduce carbon dioxide to form organic compounds. That is, they evolved the ability to photosynthesize. Hydrogen, derived from various sources, was captured using light-powered reactions to reduce fixed carbon dioxide in the Calvin cycle. The group of Gram-negative bacteria that gave rise to cyanobacteria used water as the hydrogen source and released O2 as a waste product.
Eventually, the amount of photosynthetic oxygen built up in some environments to levels that posed a risk to living organisms, since oxygen can damage many organic compounds. Various metabolic processes evolved that protected organisms from oxygen, one of which, aerobic respiration, also generated high levels of ATP. It became widely present among prokaryotes, including in a group we now call alpha-proteobacteria. Organisms that did not acquire aerobic respiration had to remain in oxygen-free environments. Originally, oxygen-rich environments were likely localized around places where cyanobacteria were active, but by about 2 billion years ago, geological evidence shows that oxygen was building up to higher concentrations in the atmosphere. Oxygen levels similar to today’s levels only arose within the last 700 million years.
Recall that the first fossils that we believe to be eukaryotes date to about 2 billion years old, so they appeared as oxygen levels were increasing. Also, recall that all extant eukaryotes descended from an ancestor with mitochondria. These organelles were first observed by light microscopists in the late 1800s, where they appeared to be somewhat worm-shaped structures that seemed to be moving around in the cell. Some early observers suggested that they might be bacteria living inside host cells, but these hypotheses remained unknown or rejected in most scientific communities.
Review Question:
Lynn Margulis and the Endosymbiotic Theory
In the late 1960s, Lynn Margulis studied the structure of cells. Mitochondria, for example, are bodies within the cell that generate the energy required for metabolism. To Margulis, they looked remarkably like bacteria. She knew that scientists had been struck by the similarity ever since the discovery of mitochondria at the end of the 1800s. Some even suggested that mitochondria began from bacteria that lived in a permanent symbiosis within the cells of animals and plants. There were parallel examples in all plant cells. Algae and plant cells have a second set of bodies (chloroplasts) that they use to carry out photosynthesis. These structures capture incoming sunlight energy and use the energy to drive biochemical reactions including the combination of water and carbon dioxide to make organic matter. Chloroplasts, like mitochondria, bear a striking resemblance to bacteria. Scientists, and Margulis in particular, became convinced that chloroplasts, like mitochondria, evolved from symbiotic bacteria — specifically, that they descended from cyanobacteria, the light-harnessing small organisms that abound in oceans and freshwater (Figure 3).
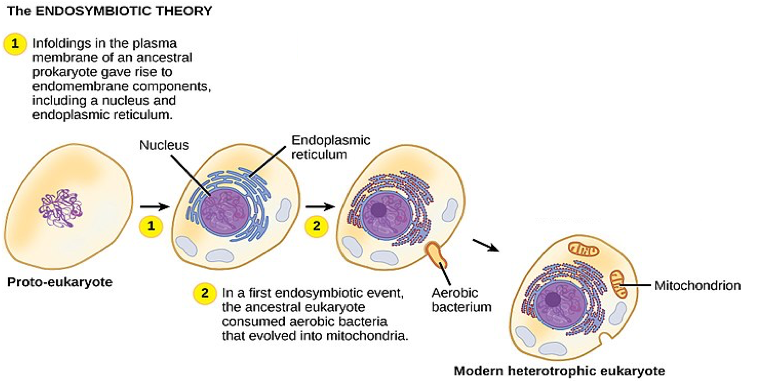
This once-revolutionary hypothesis is now widely (but not completely) accepted, with work progressing on uncovering the steps involved in this evolutionary process and the key players involved. Much still remains to be discovered about the origins of the cells that now make up the cells in all living eukaryotes.1 Broadly, it has become clear that many of our nuclear genes and the molecular machinery responsible for replication and expression appear closely related to those in Archaea. On the other hand, the metabolic organelles and genes responsible for many energy-harvesting processes had their origins in bacteria. Much remains to be clarified about how this relationship occurred; this continues to be an exciting field of discovery in biology. For instance, it is not known whether the endosymbiotic event that led to mitochondria occurred before or after the host cell had a nucleus. Such organisms would be among the extinct precursors of the last common ancestor of eukaryotes.
Review Question:
Secondary Endosymbiosis
Endosymbiosis involves one cell engulfing another to produce, over time, a co-evolved relationship in which neither cell could survive alone. The chloroplasts of red and green algae, for instance, are derived from the engulfment of a photosynthetic cyanobacterium by an early prokaryote.
This leads to the question of the possibility of a cell containing an endosymbiont itself becoming engulfed, resulting in a secondary endosymbiosis. Molecular and morphological evidence suggest that the chlorarachniophyte protists are derived from a secondary endosymbiotic event. Chlorarachniophytes are rare algae indigenous to tropical seas and sand that can be classified into the rhizarian supergroup. Chlorarachniophytes extend thin cytoplasmic strands, interconnecting themselves with other chlorarachniophytes, in a cytoplasmic network. These protists are thought to have originated when a eukaryote engulfed a green alga, the latter of which had already established an endosymbiotic relationship with a photosynthetic cyanobacterium.
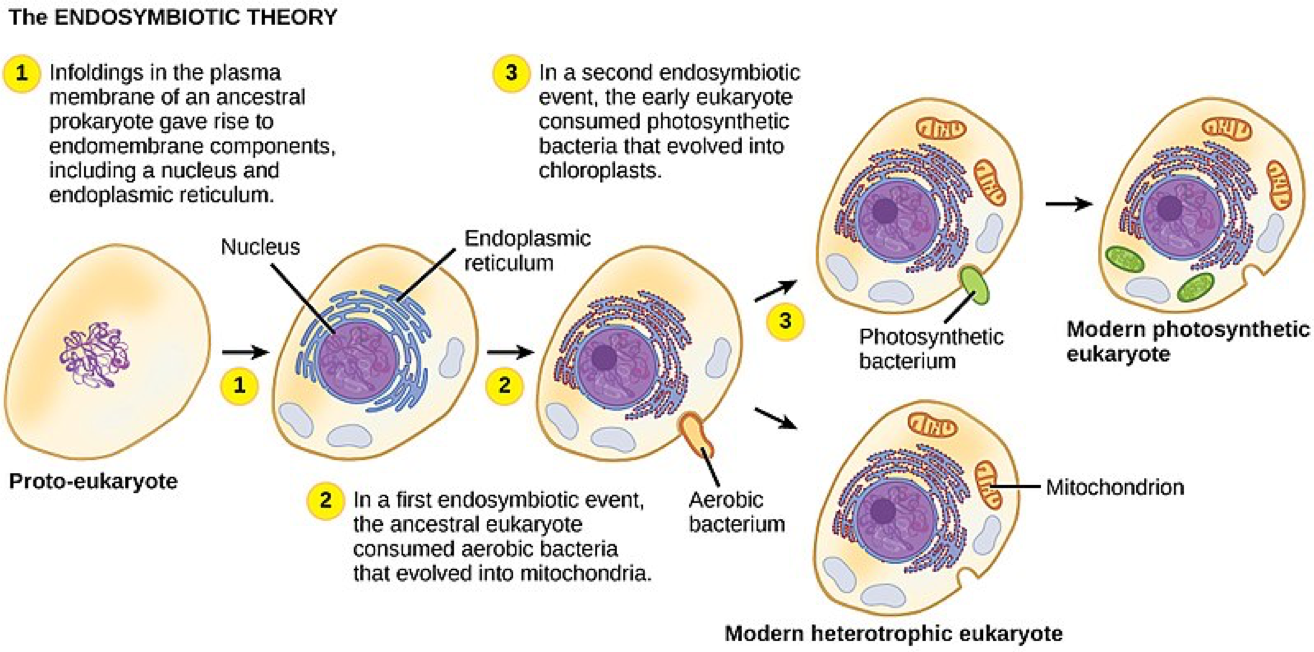
Several lines of evidence support that chlorarachniophytes evolved from secondary endosymbiosis. The chloroplasts contained within the green algal endosymbionts still are capable of photosynthesis, making chlorarachniophytes photosynthetic. The green algal endosymbiont also exhibits a stunted vestigial nucleus. In fact, it appears that chlorarachniophytes are the products of an evolutionarily recent secondary endosymbiotic event. The plastids of chlorarachniophytes are surrounded by four membranes: The first two correspond to the inner and outer membranes of the photosynthetic cyanobacterium, the third corresponds to the green alga, and the fourth corresponds to the vacuole that surrounded the green alga when it was engulfed by the chlorarachniophyte ancestor. In other lineages that involved secondary endosymbiosis, only three membranes can be identified around plastids. This is currently rectified as a sequential loss of a membrane during the course of evolution.
The process of secondary endosymbiosis is not unique to chlorarachniophytes. In fact, secondary endosymbiosis of green algae also led to euglenid protists, whereas secondary endosymbiosis of red algae led to the evolution of dinoflagellates, apicomplexans, and stramenopiles.
Summary
The oldest fossil evidence of eukaryotes is about 2 billion years old. Fossils older than this all appear to be prokaryotes. It is probable that today’s eukaryotes are descended from an ancestor that had a prokaryotic organization. The last common ancestor of today’s Eukarya had several characteristics, including cells with nuclei that divided mitotically and contained linear chromosomes where the DNA was associated with histones, a cytoskeleton and endomembrane system, and the ability to make cilia/flagella during at least part of its life cycle. It was aerobic because it had mitochondria that were the result of an aerobic alpha-proteobacterium that lived inside a host cell. Whether this host had a nucleus at the time of the initial symbiosis remains unknown. The last common ancestor may have had a cell wall for at least part of its life cycle, but more data are needed to confirm this hypothesis. Today’s eukaryotes are very diverse in their shapes, organization, life cycles, and the number of cells per individual.
End of Section Review Questions:
2) What evidence supports the theory that chlorarachniophytes evolved from secondary endosymbiosis?
3) What features do all eukaryotes share that are not found in prokaryotes?
References
1 “Endosymbiosis: Lynn Margulis” Understanding Evolution. University of California Museum of Paleontology. 22 August 2008 https://evolution.berkeley.edu/evolibrary/article/history_24
OpenStax, Biology. OpenStax CNX. Nov 7, 2018 http://cnx.org/contents/185cbf87-c72e-48f5-b51e-f14f21b5eabd@11.6. 23.1 Eukaryotic Origins
Image Attributions
Figure 1. Image created and provided by D. Jennings
Figure 2. Image courtesy of Mediran [CC BY-SA 3.0 (https://creativecommons.org/licenses/by-sa/3.0)], from Wikimedia Commons
Figure 3. Image courtesy of Phil Schatz [CC BY-SA 4.0 (https://creativecommons.org/licenses/by-sa/4.0)], via Wikimedia Commons. Modified by D. Jennings
Figure 4. Image courtesy of Phil Schatz [CC BY-SA 4.0 (https://creativecommons.org/licenses/by-sa/4.0)], via Wikimedia Commons
D, A, C, B
A, B, C, D
D
A, B, C
B, C, D