Learning Goals
By the end of this reading you should be able to:
- Describe the shared features of complex multicellular organisms
- Compare and contrast simple vs. complex multicellular organisms
- Explain the limitations to diffusion and the role of bulk flow in complex multicellular organisms
- Discuss the means by which cell adhesion, cell communication, and cell differentiation are accomplished in complex multicellular organisms
- Predict the order of acquisition of multicellular traits and the evolutionary consequences of complex multicellularity
Introduction
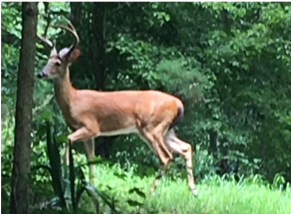
There are costs associated with multicellularity, particularly for complex multicellular organisms with differentiated reproductive tissues. In these organisms, most cells do not reproduce, instead of supporting the few that do. This requires cooperation among cells, but it creates opportunities for cells to “cheat”- to use nutrients for their own proliferation rather than the growth and reproduction of the organism as a whole. Complex multicellularity evolved at least six separate times in different eukaryotic groups; once in animal lineages, once in the green algal lineage that gave rise to land plants, twice in the fungi, once in the red algae, and once in the brown algae, producing the giant kelps that form forests in the sea.
Shared Features
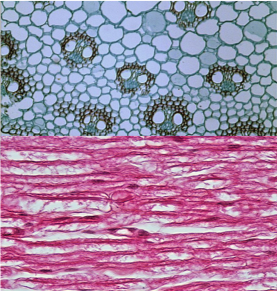
Complex multicellular organisms differ from one another in many ways, but they share three general features. (1) They have highly developed molecular mechanisms for adhesion between cells. (2) They display specialized structures that allow cells to communicate with one another. (3) They display complex patterns of cellular and tissue differentiation, guided by networks of regulatory genes. Without these features, complex multicellularity would be impossible.
For example, plants and animals both have differentiated cells and tissues with specialized functions. In plants, only some tissues photosynthesize or absorb organic molecules; other tissues transport food and oxygen through the body, and still, others generate the molecular signals that govern development. In both plants and animals, only a small subset of all cells contributes to reproduction. Because of this functional differentiation, cell or tissue loss can be lethal for the entire organism.
There is one more feature of complex multicellular organisms that is key to understanding their biology: They have a three dimensional organization, so only some cells are in direct contact with the environment. Cells that are buried within tissues, relatively far from the exterior of the organism, do not have direct access to nutrients or oxygen. Therefore, interior cells cannot grow as fast as surface cells unless there is a way to transfer resources from one part of the body to another. Similarly, interior cells do not receive signals directly from the environment, even though all cells must be able to respond to environmental signals if the organism is to grow, reproduce, and survive. Complex multicellular organisms, therefore, require mechanisms for transferring environmental signals received by cells at the body’s surface to interior cells, where genes will be activated or repressed in response. Thus growth and development in complex multicellular organisms can be defined as increasing or decreasing gene expression in response to molecular signals from surrounding cells.
Review Question:
What are the characteristics that are found in both simple colonies of cells and complex multicellular organisms?
A) the ability of cells to communicate
Diffusion and Bulk Flow
A key functional challenge of complex multicellularity is transporting food, oxygen, and molecular signals rapidly across large distances within the body. How does oxygen get from the air in your lungs into your bloodstream? How does atmospheric carbon dioxide get into leaves? How does ammonia get from seawater into the cells of seaweeds? The answer to all three questions is the same: by diffusion. But oxygen absorbed by your lungs doesn’t reach your toes by diffusion alone-it is transported actively, and in bulk, by blood pumped through your circulatory system. Water does not get from the roots to the leaves of plants by diffusion either, there are mechanisms at work to move it actively as well. For complex multicellular organisms to function, bulk flow of oxygen, nutrients, and signaling molecules, at rates and across distances far larger than can be achieved by diffusion alone is needed.
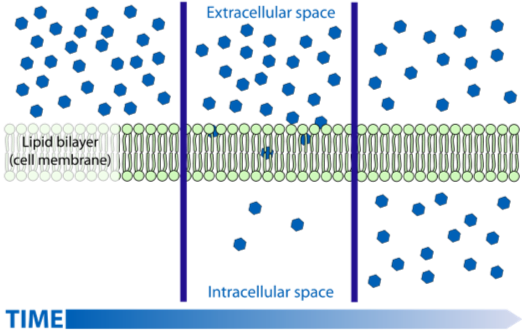
So why is diffusion effective only over short distances? Diffusion is the random motion of molecules, with net movement from areas of higher to lower concentration. The distance and speed at which molecules can diffuse depends on several factors: (1) how great the concentration difference is, large larger the differences the faster the diffusion (2) distance between concentration differences, the closer the two concentrations the faster the rate of diffusion (3) the size of the molecule, smaller molecules tend to diffuse faster than larger ones, and (4) the nature of the medium the molecule is diffusing in; molecules diffuse faster if the medium is less thick (think air vs. water). Because diffusion supplies key molecules for metabolism, it exerts a strong constraint on the size, shape, and function of cells and ultimately how eukaryotic organisms function.
Let’s use oxygen as an example. Most eukaryotes require oxygen for respiration. If the cells within a tissue must rely on diffusion for their individual oxygen supply, the thickness of the tissue is limited by the concentration difference in oxygen between the cells in the tissue and the surrounding environment. The concentration difference between the two areas depends on the amount of oxygen in the environment and the rate at which oxygen is used for respiration inside the organism. The distance between the environment and the cell that is using oxygen also plays a role.
Review Question:
Which of these would increase the rate of diffusion of a molecule?
A) a high concentration gradient between two areas involved
Organisms can achieve larger sizes by circumventing limits imposed by diffusion.
Sponges can reach overall dimensions of a meter or more, but they actually consist of only a few types of cells that line a dense network of pores and canals and so remain in close contact with circulating seawater. The large size of a sponge is therefore achieved without placing metabolically active cells at any great distance from their environment. Similarly, in jellyfish, active metabolism is confined to thin tissues that line the inner and outer surfaces of the body. Essentially, a large flat surface is folded up to produce a three-dimensional structure. An increase in the surface area of a cell and or structure increases the area that is capable of interacting with the environment and ultimately the number of needed molecules to which a cell or structure has access.
Structures specialized for bulk flow
Bulk flow is any means by which molecules move through organisms at rates beyond those possible by diffusion across a concentration gradient. In humans and other vertebrate animals, the active pumping of blood through blood vessels supplies oxygen to tissues that may be more than a meter distance from the lungs. Our lungs gather the oxygen we need for respiration, but the lung is a prime example of diffusion in action, not a means of avoiding it. Because lung tissues have a very high ratio of surface area to volume, oxygen can diffuse efficiently from the air you breathe into lung tissue. A great deal of oxygen can be taken in this way, but how does it get from the lungs to our brains or toes? The distances are far too large for diffusion to be effective.
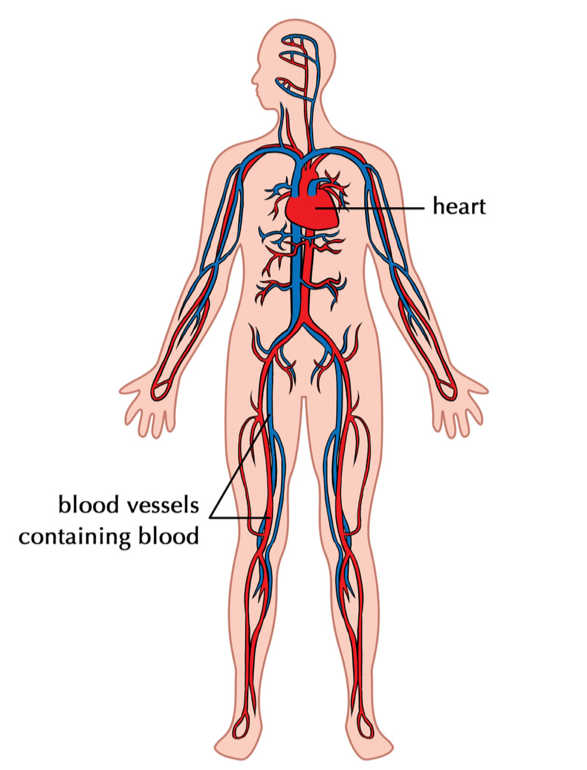
The answer is that oxygen binds to molecules of hemoglobin in red blood cells and then is carried through the bloodstream to distant sites of respiration. We circumvent diffusion by actively pumping oxygen-rich blood through our bodies. Most invertebrate animals lack well-defined blood vessels instead they have other mechanisms that circulate fluids freely throughout the body cavity. Indeed, without a mechanism like bulk flow, animals could not have achieved the range of size, shape, and function familiar to us.
Complex organisms other than animals also rely on bulk flow. A redwood tree must transport water upward from its roots to leaves that may be 100 m above the soil. If plants relied on diffusion to transport water, they would be only a few millimeters tall. How, then, do they move water? Plants move water by bulk flow through a system of specialized tissues powered by the evaporation of water from leaf surfaces. Vascular plants also have specialized tissues for the transport of nutrients and signaling molecules upward and downward through roots, stems, and leaves.
Multicellular fungi transport nutrients through networks of filaments that may be meters long, relying on osmosis to pump materials from sites of absorption to sites of metabolism. The giant kelps have an internal network of tubular cells that transports molecules through a body that can be tens of meters long. In general, when some cells within an organism are buried within tissues, far from the external environment, bulk flow is required to supply those cells with molecules needed for metabolism.
Other requirements for complex multicellular life
In addition to a means to move needed molecules over long distances and in sufficient concentration, there are three other general requirements for complex multicellular life: (1)Cells must stick together; (2) they must communicate with one another, and (3) they must participate in a network of genetic interactions that regulates cell division and differentiation. Once these are in place, the stage is set for the evolution of specialized tissues and organs in complex multicellular organisms.
Adhesion between cells
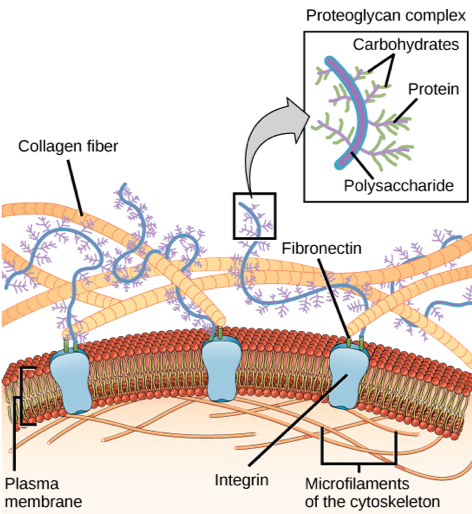
If a fertilized egg is to develop into a complex multicellular organism, it must divide many times, and the cells produced from those divisions must stick together. In addition, they must retain a specific spatial relationship with one another in order for the developing organism to function. Most animal cells release materials into the extracellular space. The primary components of these materials are proteins, and the most abundant protein is collagen. Collagen fibers are interwoven with carbohydrate-containing protein molecules called proteoglycans. Some cells attach themselves to this matrix by means of other transmembrane proteins called integrins and transmembrane proteins, especially cadherins, can also be involved in the formation of molecular attachments between cells. The extracellular matrix also allows the cells within the tissue to communicate with each other.
In plants, the cell wall connects cells together into tissues. The plant cell wall is composed predominately of cellulose fibers which are embedded in a matrix of pectin and hemicellulose. These molecules allow plant cells to attach to each other to create the complex tissue structures that support the overall plant structure. Fungi also have cell walls, although they are composed of chitin rather than cellulose, that are involved in connecting the cells together into multicellular structures.
Communication between cells
In complex multicellular organisms, it is not sufficient for cells to adhere to one another. They must also be able to communicate. Communication is important during development, guiding the patterns of gene expression that differentiate cells, tissues, and organs. The functional integration of cells within tissues and tissues within organs also depends on the flow of information among cells. Cells communicate using signaling molecules (generally a protein) synthesized by one cell which binds with a receptor protein on the surface of a second cell. This interaction can essentially flip a molecular switch that activates or represses gene expression in the receptor cell’s nucleus or stimulates another response from the receiving cells. Molecular evidence indicates that many of the signaling pathways used for communication between cells in complex multicellular organisms first evolved in single-celled eukaryotes.
Thinking Question:
What function might molecular signals and receptors have had in the ancestors of complex organisms?
All cells have transmembrane receptors that respond to signals from the environment. In some cases, the signal is a molecule released by a food organism (such as the bacteria that induce simple multicellularity in choanoflagellates), and in some cases the cells sense nutrients, temperature, or oxygen level. Single celled eukaryotes also communicate with other cells within the same species, for example, to ensure that two cells can find each other to fuse in sexual reproduction. Signaling between two cells within a complex multicellular organism (plant, animal, or fungal) can be seen as a variation on this more general theme of a cell responding to other cells and the physical environment.
While all eukaryotic cells have molecular mechanisms for communication between cells, complex multicellular organisms have distinct cellular pathways for the movement of molecules from one cell to another. For example, animals more complex than sponges have gap junctions, protein channels that allow ions and signaling molecules to move from one cell into another. Gap junctions not only help cells to communicate with their neighbors, they allow targeted communication between a cell and specific cells adjacent to it.
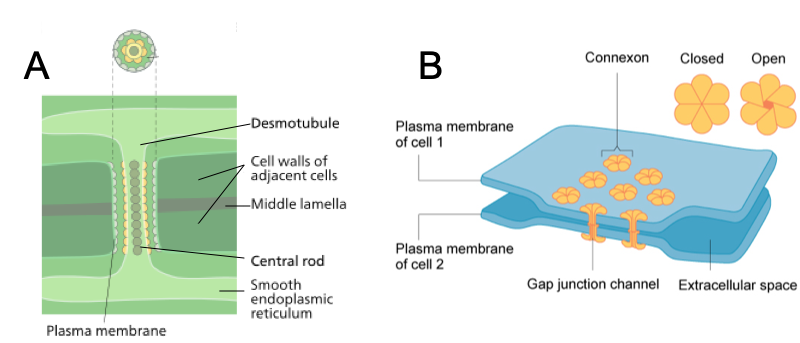
Plants, in contrast, have intercellular channels are lined by extensions of the cell membrane. Tubules running through these channels connect the endomembrane systems of the two cells. Like gap junctions, plasmodesmata permit signaling molecules to pass between cells in such a way that they can be targeted to only one or a few adjacent cells. Complex red and brown algae also have plasmodesmata, and complex fungi have pores between cells that enable communication by means of cytoplasmic flow. As similar channels do not occur in most other eukaryotic organisms, they appear to represent an important step in the evolution of complex multicellularity.
Review Question:
Genetic program for coordinated growth and cell differentiation
In multicellular organisms, growth and development is the result of molecular communication between cells. Cells have different fates depending on which genes are switched off or on, and genes are switched off and on by the molecular signals that cells receive. A signal commonly alters the production of proteins-inducing the reorganization of the cytoskeleton, for example. As a result, a stem cell may become an epithelial cell, or a muscle cell, or a neuron. This observation leads to another question: What causes the same gene to be turned on in one cell and off in another? The ultimate answer is that two cells in the same developing organism can be exposed to very different environments.
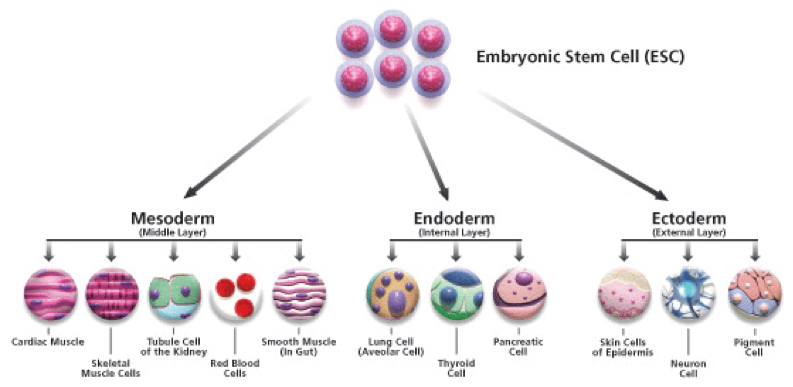
When we think about development as a process of programmed cell division and differentiation, the link to unicellular ancestors becomes clearer. Many biological innovations accompanied the evolution of complex multicellularity, but the differentiation of distinct cell types is not one of them. Many unicellular organisms have life cycles in which different cell types alternate in time, depending on environmental conditions. For example, if we experimentally starve dinoflagellate cells, two cells undergo sexual fusion to form morphologically and physiologically distinct resting cells protected by thick walls. That is, a nutrient shortage induces a change in gene expression that leads to the formation of resting cells. When food becomes available again, the cells undergo a meiotic cell division to form new feeding cells. Many other single-celled eukaryotes form resting cells in response to environmental cues, especially deprivation of nutrients or oxygen.
The innovation of complex multicellularity was to differentiate cells in space instead of time. In a three-dimensional multicellular organism, only surface cells are in direct contact with the outside environment. Interior cells are exposed to a different physical and chemical environment because nutrients, oxygen, and the light become less abundant with increasing depth within tissues. In effect, there is a gradient of environmental signals within multicellular organisms. We might, therefore, hypothesize that in the earliest organisms with three-dimensional multicellularity, a nutrient or oxygen gradient triggered oxygen- or nutrient-starved interior cells to differentiate, much as happens to trigger the formation of resting cells in their single-celled relatives. With increasing genetic control of cellular responses to signaling gradients, the seeds of complex development were sown.
Bulk flow, which transports nutrients, oxygen, and water within complex multicellular organisms, also carries developmental signals. Signals carried by bulk flow can travel far greater distances through the body than signals transmitted by diffusion alone. For example, in animals the endocrine system releases hormones directly into the bloodstream, enabling them to affect cells far from those within which they formed. Thus, the sex hormones estrogen and testosterone are synthesized in reproductive organs but regulate development throughout the body, contributing to the differences between males and females. In this way, signals carried by bulk flow can induce the formation of distinct cell types and tissues along the path of signal transport.
Review Question:
What is considered to be the greatest factor that leads to cell differentiation within complex multicellular organisms?
A) the lack of oxygen in internal spaces
B) the differing environments the cells are found in
C) the three-dimensional structure of the organism
Summary
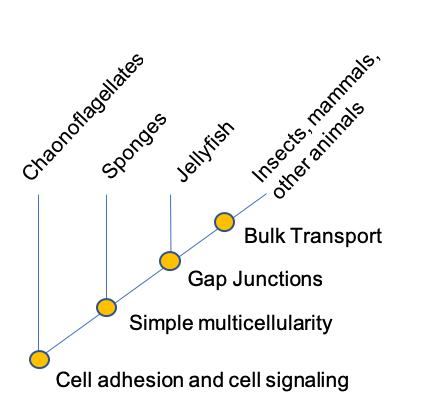
End of Section Review Questions:
3) How does cell differentiation differ between unicellular and complex multicellular organisms?A) Unicellular organisms do not exhibit cell differentiation
B) Multicellular organisms have a spatial differentiation of cells
C) Unicellular organisms have a spatial differentiation of cells
References
Image Attribution
Extra: Review of Cellular Connections
Animal cell connections: A tight junction is a watertight seal between two adjacent animal cells. The cells are held tightly against each other by proteins (predominantly two proteins called claudins and occludins). This tight adherence prevents materials from leaking between the cells; tight junctions are typically found in epithelial tissues that line internal organs and cavities and comprise most of the skin. Also found only in animal cells are desmosomes, which act like spot welds between adjacent epithelial cells. Short proteins called cadherins in the plasma membrane connect to intermediate filaments to create desmosomes. The cadherins join two adjacent cells together and maintain the cells in a sheet-like formation in organs and tissues that stretch, like the skin, heart, and muscles. Gap junctions in animal cells are like plasmodesmata in plant cells in that they are channels between adjacent cells that allow for the transport of ions, nutrients, and other substances that enable cells to communicate. Structurally, however, gap junctions and plasmodesmata differ. Gap junctions develop when a set of six proteins (called connexins) in the plasma membrane arrange themselves in an elongated donut-like configuration called a connexon. When the pores (“doughnut holes”) of connexons in adjacent animal cells align, a channel between the two cells forms. Gap junctions are particularly important in cardiac muscle: The electrical signal for the muscle to contract is passed efficiently through gap junctions, allowing the heart muscle cells to contract in tandem.
A, C
A, C
gap junctions
B
A, B
A, D, C, B
B