How do genes influence development?
Learning Objectives
- Describe genetic components of conception
- Describe genes and their importance in genetic inheritance
- Describe chromosomal abnormalities
- Explain the value of prenatal testing
- Describe the interaction between genetics and the environment
- Compare monozygotic and dizygotic twins
Heredity and Chromosomes
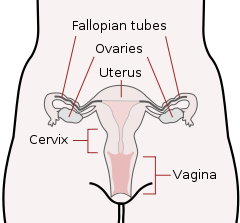
Gametes
There are two types of sex cells or gametes involved in reproduction: the male gametes, or sperm, and female gametes, or ova. The male gametes are produced in the testes through a process called spermatogenesis, which begins at about 12 years of age. The female gametes, which are stored in the ovaries, are present at birth but are immature. Each ovary contains about 250,000 ova but only about 400 of these will become mature eggs (Mackon & Fauser, 2000; Rome, 1998). Beginning at puberty, one ovum ripens and is released about every 28 days, a process called oogenesis.
After the ovum or egg ripens and is released from the ovary, it is drawn into the fallopian tube and in 3 to 4 days, reaches the uterus. It is typically fertilized in the fallopian tube and continues its journey to the uterus. At ejaculation, millions of sperm are released into the vagina, but only a few reach the egg and typically, only one fertilizes the egg. Once a single sperm has entered the wall of the egg, the wall becomes hard and prevents other sperm from entering. After the sperm has entered the egg, the tail of the sperm breaks off and the head of the sperm, containing the genetic information from the father, unites with the nucleus of the egg. As a result, a new cell is formed. This cell, containing the combined genetic information from both parents, is referred to as a zygote.
Chromosomes
While other normal human cells have 46 chromosomes (or 23 pairs), gametes contain 23 chromosomes. Chromosomes are long threadlike structures found in a cell nucleus that contains genetic material known as deoxyribonucleic acid (DNA). DNA is a helix-shaped molecule made up of nucleotide base pairs [adenine (A), guanine (G), cytosine (C), and thymine (T)]. In each chromosome, sequences of DNA make up genes that control or partially control a number of visible characteristics, known as traits, such as eye color, hair color, and so on. A single gene may have multiple possible variations or alleles. An allele is a specific version of a gene. So, a given gene may code for the trait of hair color, and the different alleles of that gene affect which hair color an individual has.
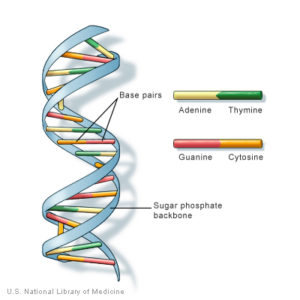
In a process called meiosis, segments of the chromosomes from each parent form pairs, and genetic segments are exchanged as determined by chance. Because of the unpredictability of this exchange, the likelihood of having offspring that are genetically identical (and not twins) is one in trillions (Gould & Keeton, 1997). Genetic variation is important because it allows a species to adapt so that those who are better suited to the environment will survive and reproduce, which is an important factor in natural selection.
Genotypes and Phenotypes
When a sperm and egg fuse, their 23 chromosomes pair up and create a zygote with 23 pairs of chromosomes. Therefore, each parent contributes half the genetic information carried by the offspring; the resulting physical characteristics of the offspring (called the phenotype) are determined by the interaction of genetic material supplied by the parents (called the genotype). A person’s genotype is the genetic makeup of that individual. Phenotype, on the other hand, refers to the individual’s inherited and observable physical and psychological characteristics, like their hair color or personality
Look in the mirror. What do you see, your genotype or your phenotype? What determines whether or not genes are expressed? Actually, this is quite complicated. Some features follow the additive pattern which means that many different genes contribute to a final outcome. Height and skin tone are examples. In other cases, a gene might either be turned on or off depending on several factors, including the gene with which it is paired or the inherited epigenetic tags.
Link to Learning
Visit the webpage “What are DNA and Genes?” from the University of Utah to better understand DNA and genes, then watch the video “What is Inheritance?” to learn how the genes from parents pass on genetic information to their children.
Determining the Sex of the Child
Twenty-two of those chromosomes from each parent are similar in length to a corresponding chromosome from the other parent. However, the remaining chromosome looks like an X or a Y. Half of the male’s sperm contains a Y chromosome and half contain an X. All of the ova contain X chromosomes. If the child receives the combination of XY, the child will be genetically male. If it receives the XX combination, the child will be genetically female.
Many potential parents have a clear preference for having a boy or a girl and would like to determine the sex of the child. Through the years, a number of tips have been offered for potential parents to maximize their chances of having either a son or daughter as they prefer. However, there is not much scientific evidence to back these claims. Today, however, there is new technology available called sperm sorting that makes it possible to isolate sperm containing either an X or a Y, depending on the preference, and use that sperm to fertilize a mother’s egg. Preimplantation genetic diagnosis (PGD) could also be used to select only embryos of the desired sex to be implanted during in-vitro fertilization (IVF). However, these methods are controversial and both fertility centers and medical organizations discourage it if there is no real medical reason to select gender.
Genetic Variation and Inheritance
Genetic variation, the genetic difference between individuals, is what contributes to a species’ adaptation to its environment. In humans, genetic variation begins with an egg, several million sperm, and fertilization. The egg and the sperm each contain 23 chromosomes, which make up our genes. A single gene may have multiple possible variations or alleles (a specific version of a gene), resulting in a variety of combinations of inherited traits.
Genetic inheritance of traits for humans is based upon Gregor Mendel’s model of inheritance. For genes on an autosome (any chromosome other than a sex chromosome), the alleles and their associated traits are autosomal dominant or autosomal recessive. In this dominant-recessive model, some genes are considered dominant because they will be expressed. Others, termed recessive, are only expressed in the absence of a dominant gene. They are less influential than the dominant gene. Some characteristics which were once thought of as dominant-recessive, such as eye color, are now believed to be a result of the interaction between several genes (McKusick, 1998). Dominant traits include curly hair, facial dimples, normal vision, and dark hair. Recessive characteristics include red hair, pattern baldness, and nearsightedness.
Sickle cell anemia is an autosomal recessive disease; Huntington’s disease is an autosomal dominant disease. Other traits are a result of partial dominance or co-dominance in which both genes are influential. For example, if a person inherits both recessive genes for cystic fibrosis, the disease will occur. But if a person has only one recessive gene for the disease, the person would be a carrier of the disease.
In this example, we will call the normal gene “N,” and the gene for cystic fibrosis “c.” The normal gene is dominant, which means that having the dominant allele either from one parent (Nc) or both parents (NN) will always result in the phenotype associated with the dominant allele. When someone has two copies of the same allele, they are said to be homozygous for that allele. When someone has a combination of alleles for a given gene, they are said to be heterozygous. For example, cystic fibrosis is a recessive disease which means that an individual will only have the disease if they are homozygous for that recessive allele (cc).
Imagine that a woman who is a carrier of the cystic fibrosis gene has a child with a man who also is a carrier of the same disease. What are the odds that their child would inherit the disease? Both the woman and the man are heterozygous for this gene (Nc). We can expect the offspring to have a 25% chance of having cystic fibrosis (cc), a 50% chance of being a carrier of the disease (Nc), and a 25% chance of receiving two normal copies of the gene (NN).
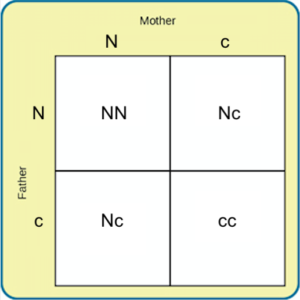
Where do harmful genes that contribute to diseases like cystic fibrosis come from? Gene mutations provide one source of harmful genes. A mutation is a sudden, permanent change in a gene. While many mutations can be harmful or lethal, once in a while a mutation benefits an individual by giving that person an advantage over those who do not have the mutation. Recall that the theory of evolution asserts that individuals best adapted to their particular environments are more likely to reproduce and pass on their genes to future generations. For this process to occur, there must be competition—more technically, there must be variability in genes (and resultant traits) that allow for variation in adaptability to the environment. If a population consisted of identical individuals, then any dramatic changes in the environment would affect everyone in the same way, and there would be no variation in selection. In contrast, diversity in genes and associated traits allow some individuals to perform slightly better than others when faced with environmental change. This creates a distinct advantage for individuals best suited for their environments in terms of successful reproduction and genetic transmission.
Link to Learning
Visit the Cystic Fibrosis Foundation to learn more about cystic fibrosis and learn how a mutation in DNA leads to the disease.
Chromosomal Abnormalities and Genetic Testing
Chromosomal Abnormalities
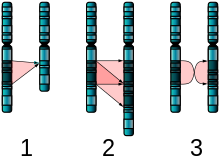
A chromosomal abnormality occurs when a child inherits too many or too few chromosomes. The most common cause of chromosomal abnormalities is the age of the mother. A 20-year-old woman has a 1 in 800 chance of having a child with a common chromosomal abnormality. A woman of 44, however, has a one in 16 chance. It is believed that the problem occurs when the ovum is ripening prior to ovulation each month. As the mother ages, the ovum is more likely to suffer abnormalities at this time.
Another common cause of chromosomal abnormalities occurs because the gametes do not divide evenly when they are forming. Therefore, some cells have more than 46 chromosomes. In fact, it is believed that close to half of all zygotes have an odd number of chromosomes. Most of these zygotes fail to develop and are spontaneously aborted by the body. If the abnormal number occurs on pair # 21 or # 23, however, the individual may have certain physical or other abnormalities.
An altered chromosome structure may take several different forms, and result in various disorders or malignancies:
- Deletions: A portion of the chromosome is missing or deleted. Known disorders in humans include Wolf-Hirschhorn syndrome, which is caused by partial deletion of the short arm of chromosome 4; and Jacobsen syndrome also called the terminal 11q deletion disorder.
- Duplications: A portion of the chromosome is duplicated, resulting in extra genetic material. Known human disorders include Charcot-Marie-Tooth disease type 1A, which may be caused by duplication of the gene encoding peripheral myelin protein 22 (PMP22) on chromosome 17.
Figure 7. The two major two-chromosome mutations: insertion (1) and Translocation (2). - Translocations: A portion of one chromosome is transferred to another chromosome. There are two main types of translocations:
- Reciprocal translocation: Segments from two different chromosomes have been exchanged.
- Robertsonian translocation: An entire chromosome has attached to another at the centromere – in humans, these only occur with chromosomes 13, 14, 15, 21, and 22.
- Inversions: A portion of the chromosome has broken off, turned upside down, and reattached, therefore the genetic material is inverted.
- Insertions: A portion of one chromosome has been deleted from its normal place and inserted into another chromosome.
- Rings: A portion of a chromosome has broken off and formed a circle or ring. This can happen with or without loss of genetic material.
- Isochromosome: Formed by the mirror image copy of a chromosome segment including the centromere.
One of the most common chromosomal abnormalities is on pair # 21. Trisomy 21 occurs when there are three rather than two chromosomes on #21. A person with Down syndrome has distinct facial features, intellectual disability, and oftentimes heart and gastrointestinal disorders. Symptoms vary from person to person and can range from mild to severe. With early intervention, the life expectancy of persons with Down syndrome has increased in recent years. Keep in mind that there is as much variation in people with Down Syndrome as in most populations and those differences need to be recognized and appreciated.
When the chromosomal abnormality is on pair #23, the result is a sex-linked chromosomal abnormality. A person might have XXY, XYY, XXX, XO, or 45 or 47 chromosomes as a result. Two of the more common sex-linked chromosomal disorders are Turner syndrome and Klinefelter syndrome. Turner’s syndrome occurs in 1 of every 2,500 live female births when an ovum that lacks a chromosome is fertilized by a sperm with an X chromosome (Carroll, 2007). The resulting zygote has an XO composition. Fertilization by a Y sperm is not viable. Turner syndrome affects cognitive functioning and sexual maturation. The external genitalia appear normal, but breasts and ovaries do not develop fully and the woman does not menstruate. Turner’s syndrome also results in short stature and other physical characteristics. Klinefelter syndrome (XXY) occurs in 1 out of 700 live male births and results when an ovum containing an extra X chromosome is fertilized by a Y sperm. The Y chromosome stimulates the growth of male genitalia, but the additional X chromosome inhibits this development. An individual with Klinefelter syndrome has some breast development, infertility (this is the most common cause of infertility in males), and has low levels of testosterone.
Prenatal Testing
Prenatal testing consists of prenatal screening and prenatal diagnosis, which are aspects of prenatal care that focus on detecting problems with the pregnancy as early as possible. These may be anatomic and physiologic problems with the health of the zygote, embryo, or fetus, either before gestation even starts or as early in gestation as practical. Prenatal screening focuses on finding problems among a large population with affordable and noninvasive methods. The most common screening procedures are routine ultrasounds, blood tests, and blood pressure measurement. Prenatal diagnosis focuses on pursuing additional detailed information once a particular problem has been found, and can sometimes be more invasive.
Screening can detect problems such as neural tube defects, anatomical defects, chromosome abnormalities, and gene mutations that would lead to genetic disorders and birth defects, such as spina bifida, cleft palate, Downs Syndrome, Tay–Sachs disease, sickle cell anemia, thalassemia, cystic fibrosis, muscular dystrophy, and fragile X syndrome. Some tests are designed to discover problems that primarily affect the health of the mother, such as PAPP-A to detect pre-eclampsia or glucose tolerance tests to diagnose gestational diabetes. Screening can also detect anatomical defects such as hydrocephalus, anencephaly, heart defects, and amniotic band syndrome.
Common prenatal diagnosis procedures include amniocentesis and chorionic villus sampling. Because of the miscarriage and fetal damage risks associated with amniocentesis and CVS procedures, many women prefer to first undergo screening so they can find out if the fetus’ risk of birth defects is high enough to justify the risks of invasive testing. Screening tests yield a risk score which represents the chance that the baby has the birth defect; the most common threshold for high-risk is 1:270. A risk score of 1:300 would, therefore, be considered low-risk by many physicians. However, the trade-off between the risk of birth defects and risk of complications from invasive testing is relative and subjective; some parents may decide that even a 1:1000 risk of birth defects warrant an invasive test while others wouldn’t opt for an invasive test even if they had a 1:10 risk score.
There are three main purposes of prenatal diagnosis: (1) to enable timely medical or surgical treatment of a condition before or after birth, (2) to give the parents the chance to abort a fetus with the diagnosed condition, and (3) to give parents the chance to prepare psychologically, socially, financially, and medically for a baby with a health problem or disability, or for the likelihood of stillbirth. Having this information in advance of birth means that healthcare staff, as well as parents, can better prepare themselves for the delivery of a child with a health problem. For example, Down Syndrome is associated with cardiac defects that may need intervention immediately upon birth.
The American College of Obstetricians and Gynecologists (ACOG) guidelines currently recommend that all pregnant women, regardless of age, be offered invasive testing to obtain a definitive diagnosis of certain birth defects. Therefore, most physicians offer diagnostic testing to all their patients, with or without prior screening, and let the patient decide.
Behavioral Genetics
Behavioral geneticists study how individual differences arise, in the present, through the interaction of genes and the environment. When studying human behavior, behavioral geneticists often employ twin and adoption studies to research questions of interest. Twin studies compare the rates that a given behavioral trait is shared among identical and fraternal twins; adoption studies compare those rates among biologically related relatives and adopted relatives. Both approaches provide some insight into the relative importance of genes and environment for the expression of a given trait.
The evolutionary perspective encompasses one of the fastest-growing areas within the field of lifespan development: behavioral genetics. Behavioral genetics is a field of scientific research that uses genetic methods to investigate the nature and origins of individual differences in behavior and studies the effects of heredity on behavior. Behavioral geneticists strive to understand how we might inherit certain behavioral traits and how the environment influences whether we actually displayed those traits. It also considers how genetic factors may influence psychological disorders such as schizophrenia, depression, and substance abuse. They use a measure called heritability to estimate how well differences in person’s genes account for differences in their traits in a given population.
Nature or Nurture?
For decades, scholars have carried on the “nature/nurture” debate. For any particular feature, those on the “nature” side would argue that heredity plays the most important role in bringing about that feature. Those on the “nurture” side would argue that one’s environment is most significant in shaping the way we are. This debate continues in questions about what makes us masculine or feminine (Lippa, 2002), concerns about vision (Mutti et al., 1996), and many other developmental issues.
Most scholars agree that there is a constant interplay between the two forces. It is difficult to isolate the root of any single behavior as a result solely of nature or nurture, and most scholars believe that even determining the extent to which nature or nurture impacts a human feature is difficult to answer. In fact, almost all human features are polygenic (a result of many genes) and multifactorial (a result of many factors, both genetic and environmental). It is as if one’s genetic make-up sets up a range of possibilities, which may or may not be realized depending upon one’s environmental experiences. For instance, a person might be genetically predisposed to develop diabetes, but the person’s lifestyle may help bring about the disease.
When you think about your own family history, it is easy to see that there are certain personality traits, behavioral characteristics, and medical conditions that are more common than others. This is the reason that doctors ask you about your family medical history. While genetic predisposition is important to consider, there are some family members who, for a variety of reasons, seemed to defy the odds of developing these conditions. These differences can be explained in part by the effect of epigenetic (above the genome) changes.
The Epigenetic Framework
The term “epigenetic” has been used in developmental psychology to describe psychological development as the result of an ongoing, bi-directional interchange between heredity and the environment. Gottlieb (1998; 2000; 2002) suggests an analytic framework for the nature/nurture debate that recognizes the interplay between the environment, behavior, and genetic expression. This bidirectional interplay suggests that the environment can affect the expression of genes just as genetic predispositions can impact a person’s potentials. Likewise, environmental circumstances can trigger symptoms of a genetic disorder. For example, a person predisposed genetically for type 2 diabetes may trigger the disease through poor diet and little exercise.
The developmental psychologist Erik Erikson wrote of an epigenetic principle in his book Identity: Youth and Crisis (1968), encompassing the notion that we develop through an unfolding of our personality in predetermined stages, and that our environment and surrounding culture influence how we progress through these stages. This biological unfolding in relation to our socio-cultural settings is done in stages of psychosocial development, where “progress through each stage is in part determined by our success, or lack of success, in all the previous stages.”
In typical human families, children’s biological parents raise them, so it is very difficult to know whether children act like their parents due to genetic (nature) or environmental (nurture) reasons. Nevertheless, despite our restrictions on setting up human-based experiments, we do see real-world examples of nature-nurture at work in the human sphere—though they only provide partial answers to our many questions. The science of how genes and environments work together to influence behavior is called behavioral genetics. The easiest opportunity we have to observe this is the adoption study. When children are put up for adoption, the parents who give birth to them are no longer the parents who raise them. Children aren’t assigned to random adoptive parents in order to suit the particular interests of a scientist but adoption still tells us some interesting things, or at least confirms some basic expectations. For instance, if the biological child of tall parents were adopted into a family of short people, do you suppose the child’s growth would be affected? What about the biological child of a Spanish-speaking family adopted at birth into an English-speaking family? What language would you expect the child to speak? And what might these outcomes tell you about the difference between height and language in terms of nature-nurture?
Monozygotic and Dizygotic Twins
Another option for observing nature-nurture in humans involves twin studies. To analyze nature-nurture using twins, we compare the similarity of monozygotic and dizygotic pairs. Monozygotic twins occur when a single zygote or fertilized egg splits apart in the first two weeks of development. The result is the creation of two separate but genetically identical offspring. About one-third of twins are monozygotic twins. Monozygotic twins occur in birthing at a rate of about 3 in every 1000 deliveries worldwide (about 0.3% of the world population). Monozygotic twins are genetically nearly identical and they are always the same sex unless there has been a mutation during development. The children of monozygotic twins test genetically as half-siblings (or full siblings, if a pair of monozygotic twins reproduces with another pair of identical twins or with the same person), rather than first cousins.
Sometimes two eggs or ova are released and fertilized by two separate sperm. The result is dizygotic or fraternal twins. About two-thirds of twins are dizygotic. These two individuals share the same amount of genetic material as would any two children from the same mother and father. Older mothers are more likely to have dizygotic twins than are younger mothers and couples who use fertility drugs are also more likely to give birth to dizygotic twins. Consequently, there has been an increase in the number of fraternal twins in recent years (Bortolus et al., 1999). In vitro fertilization (IVF) techniques are more likely to create dizygotic twins. For IVF deliveries, there are nearly 21 pairs of twins for every 1,000.
In the uterus, a majority of monozygotic twins (60–70%) share the same placenta but have separate amniotic sacs. The placenta is a temporary organ that connects the developing fetus via the umbilical cord to the uterine wall to allow nutrient uptake, thermo-regulation, waste elimination, and gas exchange via the mother’s blood supply. The amniotic sac (also called the bag of waters or the membranes), is a thin but tough transparent pair of membranes that hold a developing embryo (and later fetus) until shortly before birth. In 18–30% of monozygotic twins each fetus has a separate placenta and a separate amniotic sac. A small number (1–2%) of monozygotic twins share the same placenta and amniotic sac. Fraternal twins each have their own placenta and own amniotic sac.

Monozygotic (one egg/identical) twins can be categorized into four types depending on the timing of the separation and duplication of cells. Various types of chorionicity and amniosity (how the baby’s sac looks) in monozygotic twins are a result of when the fertilized egg divides. This is known as placentation.
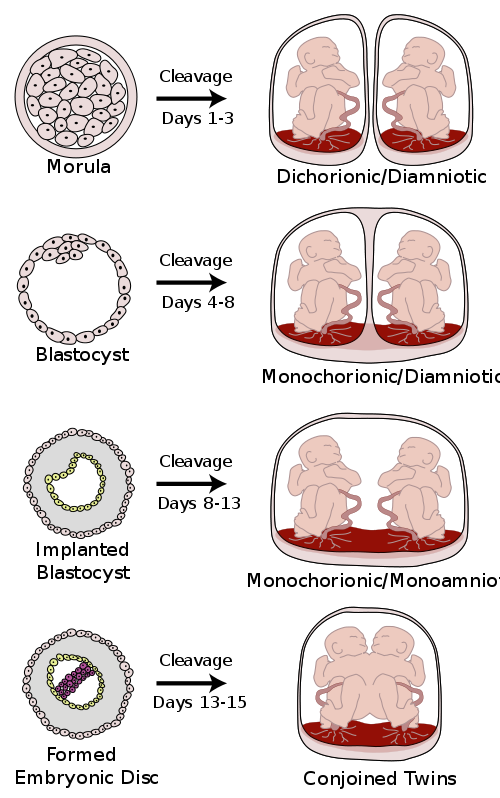
Conjoined twins
Conjoined twins are monozygotic twins whose bodies are joined together during pregnancy. This occurs when the zygote starts to split after day 12 following fertilization and fails to separate completely. This condition occurs in about 1 in 50,000 human pregnancies. Most conjoined twins are now evaluated for surgery to attempt to separate them into separate functional bodies. The degree of difficulty rises if a vital organ or structure is shared between twins, such as the brain, heart, or liver.
Vanishing twins
Researchers suspect that as many as 1 in 8 pregnancies start out as multiples, but only a single fetus is brought to full term because the other fetus has died very early in the pregnancy and has not been detected or recorded. Early obstetric ultrasonography exams sometimes reveal an “extra” fetus, which fails to develop and instead disintegrates and vanishes in the uterus. There are several reasons for the “vanishing” fetus, including it being embodied or absorbed by the other fetus, placenta or the mother. This is known as vanishing twin syndrome. Also, in an unknown proportion of cases, two zygotes may fuse soon after fertilization, resulting in a single chimeric embryo, and, later, fetus.
Twin Studies
Using the features of height and spoken language as examples, let’s take a look at how nature and nurture apply: identical twins, unsurprisingly, are almost perfectly similar for height. The heights of fraternal twins, however, are like any other sibling pairs: more similar to each other than to people from other families, but hardly identical. This contrast between twin types gives us a clue about the role genetics plays in determining height.
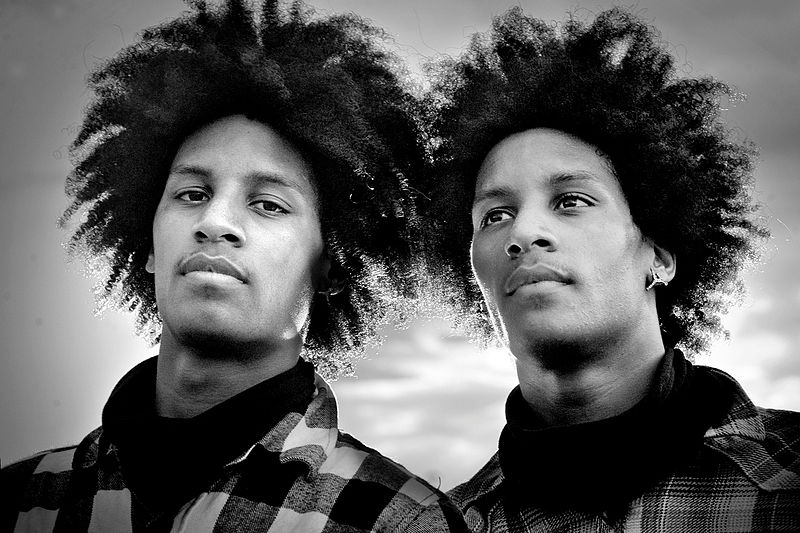
Now consider spoken language. If one identical twin speaks Spanish at home, the co-twin with whom she is raised almost certainly does too. But the same would be true for a pair of fraternal twins raised together. In terms of spoken language, fraternal twins are just as similar as identical twins, so it appears that the genetic match of identical twins doesn’t make much difference.
Twin and adoption studies are two instances of a much broader class of methods for observing nature-nurture called quantitative genetics, the scientific discipline in which similarities among individuals are analyzed based on how biologically related they are. We can do these studies with siblings and half-siblings, cousins, and twins who have been separated at birth and raised separately (Bouchard et al., 1990). Such twins are very rare and play a smaller role than is commonly believed in the science of nature-nurture, or with entire extended families (Plomin et al., 2012).
It would be satisfying to be able to say that nature-nurture studies have given us conclusive and complete evidence about where traits come from, with some traits clearly resulting from genetics and others almost entirely from environmental factors, such as child-rearing practices and personal will; but that is not the case. Instead, everything has turned out to have some footing in genetics. The more genetically-related people are, the more similar they are—for everything: height, weight, intelligence, personality, mental illness, etc. Sure, it seems like common sense that some traits have a genetic bias. For example, adopted children resemble their biological parents even if they have never met them, and identical twins are more similar to each other than are fraternal twins. And while certain psychological traits, such as personality or mental illness (e.g., schizophrenia), seem reasonably influenced by genetics, it turns out that the same is true for political attitudes, how much television people watch (Plomin et al., 1990), and whether or not they get divorced (McGue & Lykken, 1992).