Chapters
3
Overview
The human body is awash in a sea of microbes. To prevent invasion by foreign pathogens and/or ‘non-self’, the body has evolved complementary and overlapping defense mechanisms, or barriers, as depicted below (Figure 35).
Figure 35: Barriers against pathogens and non-self
The defense mechanisms to pathogens and non-self include:
- Physical barriers
- Inflammatory response
- Innate and acquired immune responses
In a nutshell, the function of these defense mechanisms is to preserve homeostasis by protecting the body from ‘non-self’, or any material which may be considered foreign to the body and which may cause disease. Non-self can include microbial pathogens, organic molecules such as proteins, as well as non-organic material such as asbestos or coal dust. Non-self can also extend to cancerous cells and those transformed by viruses. In this unit, we will discuss each mechanism separately, although as alluded to above, each of these systems to varying degrees overlap functionally and physically. By the end of this unit, you should have a clear understanding of the overall function of the mechanisms protecting the human body from non-self. Disordered function of any one of these systems can result in pathology, and we will discuss specific examples of disease states that result from either hyper- or hypo-functioning defense mechanisms.
Learning Outcomes
Completion of this chapter’s readings and learning activities will enable you to:
3.1: Discuss physical barriers which prevent the entry of ‘non-self’
3.2: Understand the signs, symptoms, and effectors of the inflammatory response
3.3 Describe the cellular and humoral effectors of the innate immune response
3.4: Describe the cellular and humoral effectors of the acquired (adaptive) immune response
3.5 Distinguish between hypersensitivity reactions, autoimmunity, and immune deficiencies
Key Concepts
- Physical barriers are first line of defense
- The inflammatory response is primarily a vascular process that has evolved to isolate microorganisms
- Cytokines regulate inflammatory and immune responses, and include interleukins and interferons
- The inflammatory and innate immune response are rapid, relatively non-specific defense mechanisms against ‘non-self’
- Toll-like receptors (TLRs) are pattern recognition molecules which facilitate the recognition of bacterial and viral pathogens, as well as molecules released by damaged cells
- The acquired or adaptive immune response utilizes antibodies, MHC II receptors and T cell receptors to recognize specific pathogens
- B cells synthesize antibodies
- Antigen presenting cells are part of the innate and acquired immune responses, and include dendritic cells, Langerhans cells, and B cells
- T cells include several subclasses which help regulate the acquired immune response
- Hypersensitivity reactions are exaggerated responses to either self or non-self molecules
Learning Units
Unit 3.2 The Inflammatory Response
Unit 3.3 The Innate Immune Response
Unit 3.4 The Acquired (Adaptive) Immune Response
Unit 3.5 Hypersensitivity, Autoimmunity, Immune Deficiencies
Unit 3.1 Physical Barriers to Non-Self
Physical barriers (examples in Figure 36) prevent pathogens from gaining access to intracellular, intravascular, and/or interstitial spaces.
Figure 36: Physical barriers to pathogens and non-self
We can list these physical barriers as follows:
I. Tears covering the corneal epithelium of the eye
II. Mucous barriers over epithelial such as the intestinal and respiratory tract
III. Tight and occluding cell-cell junctions
IV. Stratified, cornified epithelia such as the skin
V. Others (not depicted) such as stomach acidity
Mucous provides a microenvironment on the apical surfaces of the respiratory and gastrointestinal tracts which traps pathogens, in addition to keeping the cell surface moist. Mucous, tears, and other secretions also are usually rich in antibacterial factors (eg: defensins, cryptidins), proteases (eg: lysozyme), and antibodies (eg: immunoglobulin E) which inhibit bacterial growth.
The external and internal environments of the body are physically separated cell-cell junctions such as tight and occluding junctions. These not only inhibit bacterial translocation; they also prevent large molecules such as proteins from gaining access to inner body compartments.
Finally, the inner and outer surfaces of the body are covered by a multilayered (stratified) epithelium. Over the skin, the uppermost layer of (dead) cells is enriched in keratin (cornified). Parts of the esophagus and vagina are also covered by a stratified epithelium, but this is not cornified. Instead, antibacterial secretions help to protect from pathogenic invasion.
When any of the barriers become compromised, pathogens can gain entry, resulting in infection and inflammation.
Self-Study Question:
Unit 3.2 The Inflammatory Response
The inflammatory response, which can be considered to be part of the innate immune response, functions to isolate and destroy pathogens. A key point to remember is that many diseases are disorders of inflammation: arthritis, eczema, heart disease, emphysema, and kidney disease all include elements of inflammation. Thus, it is important for us to have an understanding of both the signs and symptoms of inflammation, as well as the underlying physiology of the inflammatory response. Before doing so, we should differentiate between clinical signs and symptoms: a clinical sign is an objective, observable change; a clinical symptom is a subjective experience.
A cardinal systemic sign of inflammation is fever. The underlying pathophysiological mechanisms behind fever are varied, and may not be inflammatory in nature; however the chief cause of fever in approximately 75% of instances is pyrogenic. As depicted below (Figure 37), pyrogens, or fever-inducing molecules, include LPS (a component of gram-negative bacterial cell walls) or inflammatory cytokines such as TNF-α, which stimulate prostaglandin E (PGE) release by immune cells. PGE activates hypothalamic cells which regulate body temperature by increasing thermal energy generation. Aside from pyrogenic fever, other causes of fever can include tissue ischemia, malignancy, and drug reactions.
Figure 37: Pyrogens and fever induction
Self-Study Question:
The signs and symptoms of localized inflammation have been recognized at least since ancient Roman times, and include the following (Figure 38):
Figure 38: Local signs and symptoms of inflammation
Calor (heat), rubor (redness), dolor (pain), tumor (edema), and functio Laesa (loss of function) are hallmarks of inflammation. These may be rationalized when you remember that inflammation is essentially a vascular event; in other words, vascular hemodynamics are altered during the inflammatory response, including:
- Increased arteriolar radius, and
- Increased vascular permeability
This results in both an increase in local blood flow, and leakage of plasma proteins into the interstitial space. The former is due to the increased blood flow (hyperemia), whereas the latter is secondary to the loss of oncotic plasma proteins, especially albumin, into the interstitial space and the concomitant osmotic flow of water. The increased blood flow also overwhelms the ability of local lymphatic drainage. As depicted below (Figure 39), one cell stands out: the mast cell, which synthesizes and releases factors that cause smooth muscle relaxation, and widened gaps between the endothelial cells lining capillaries.
Figure 39: Cellular mediators of the inflammatory response
The end results of these activities are that blood flow increases through the capillaries, generating redness and warmth, while proteins and fluids leave the intravascular space, causing swelling or edema. Also depicted is the extravasation of monocytes, which become tissue macrophages, after they move through gaps in the endothelium. Other cellular effectors, including neutrophils and platelets, are part of this cascading process. Given that they play such central roles in the inflammatory process, we will expand upon the functions of mast cells later.
In addition to the cellular mediators, molecules, or humoral factors, are involved in the inflammatory response. Below is a partial list of these humoral factors, and their primary actions.
Kinins:
- Lead to formation of bradykinin from cleavage of precursors
- Increase vascular permeability, arteriolar dilation, and non-vascular smooth muscle contraction (eg: bronchial smooth muscle)
- Cause pain
- Rapidly inactivated (kininases)
Complement C1-C9:
- Assembled as a complex; forms pores in the cell membranes of microbes and cells, causing cellular lysis
- During acute inflammation, complement components cause:
- Vasodilation, vascular permeability, mast cell degranulation (C3a, C5a)
- Leukocyte chemotaxis (C5a)
- Complement also opsonizes molecules or cells, increasing phagocytosis (C3b, C3bi)
Arachidonic acid metabolites (eicosanoids):
- Prostaglandins and thromboxane
- Cause vasodilation and prolong edema
- Leukotrienes
- Chemotaxins, vasoconstrictors
- Cause increased vascular permeability and bronchospasm
Nitric Oxide
- Short-acting, soluble gas with many functions
- Produced by endothelial cells, macrophages
- Causes vascular smooth muscle relaxation and vasodilation
- Kills microbes in activated macrophages
- Counteracts platelet adhesion, aggregation, and degranulation
PAF (platelet activating factor)
- Derived from cell membrane phospholipid
- Induces vasodilation, increased vascular permeability, increases leukocyte adhesion
The Mast Cell
Mast cells are key mediators of the inflammatory response, and may be activated by a variety of factors, as evidenced by the array of receptors displayed on the cell surface. For example, receptors of the FC portion of antibodies (discussed later in this unit), toll-like receptors, histamine receptors, as well as chemokine and cytokine receptors may, when bound to their specific ligands, activate mast cells (Figure 40).
Figure 40: Mast cell receptors and inflammatory products
Chemotaxins and cytokines deserve further mention, as we will refer to the roles of these molecules throughout this unit. Cytokines are a diverse group of peptides that regulate cell signalling, and include interferons (IFNs), interleukins (ILs), and tumor necrosis factors (TNFs), to name a few. They are produced by a broad range of cells, including immune cells, fibroblasts, and epithelial cells, and play significant roles in inflammation and innate and acquired immunity. These can be either pro-inflammatory (eg: IL-1, IL-6, TNF-alpha) or anti-inflammatory (IL-4, IL-6, IL-10). Note that IL-6 can be both pro- and anti-inflammatory, depending on circumstance. Chemokines are even smaller, cytokine-like peptides that regulate the activity of leukocytes. For example, monocyte chemoattractant peptide 1 (MCP-1) attracts monocytes to leave the vascular space and enter the interstitial (between cell) space.
What occurs after mast cell activation can be loosely categorized as part of either the ‘slow’ or ‘fast’ phase of activation. During the fast phase, mast cells release membrane-bound granules (degranulate), releasing vasoactive factors which affect capillary diameter and permeability, or chemotactic factors that induce chemotaxis or cell movement of neutrophils and eosinophils. A second, slower phase of mast cell activation includes the upregulation or increase in synthesis of both of the aforementioned molecules. In addition to those we’ve already discussed, other molecules released by mast cells include vasoactive amines, histamine, serotonin, plasma proteases, and clotting enzymes
Self-Study Question:
Histamine is released by mast cells. There are also histamine receptors on mast cells.
- What is the effect of histamine binding to receptors on mast cells?
- What type of reflex is this? (Refer to Chapter 2)
- What types of histamine receptors are there, and what happens when these are activated?
Summary
The inflammatory response is primarily a vascular event, and one of the main cellular effectors of the inflammatory response is the mast cell, although other cells are involved or recruited during this process. A variety of humoral factors mediate the inflammatory response; chief among them is histamine. As we will see in Chapter 4, inflammation is also associated with some cancers, either as a causal factor or as a sign and symptom.
The efficacy of the immune response is primarily in its ability to recruit mediators such as monocytes and neutrophils which are able to phagocytose pathogens. In terms of maintaining homeostasis, another potential benefit of the inflammatory reaction is its ability to generate swelling in tissues, which may help ‘wall off’ pathogens and elicit fever. On the other hand, inflammation is a hallmark of many diseases, and when carried to its extreme, may itself cause cell, tissue, and organ damage.
As we shall see in the next sections, the inflammatory response may be considered an integrated element of immunity, and some of the key cellular effectors, including mast cells, are shared components of innate and acquired immunity.
Unit 3.3 The Innate Immune Response

The human body is a nutrient-rich environment that ideal for the growth of remarkably diverse microorganisms, many of which are pathogenic, or disease-causing. Consequently, from the moment of birth we must be capable of mounting a defense against microorganisms which elude the physical barriers discussed previously. The fundamental function of an immune response is to recognize and respond to non-self (Figure 41).
Figure 41: Basic function of the immune response
As discussed earlier, non-self is that which normally is not found in the body. For an immune response to be effective, it must be able to both recognize and respond to non-self. Under this broad definition, we can categorize two types of immunity: innate and acquired (or adaptive). As per any definition of the term ‘innate’ immunity implies that it is present at birth. Acquired immunity, by definition, develops with time and exposure. The innate and immune systems overlap with respect to some of their shared cellular and molecular mediators, but they differ fundamentally in other ways.
The innate immune system differs from that acquired later during development in several regards:
- It responds very rapidly to non-self.
- It recognizes only broad molecular patterns, and is therefore relatively non-specific.
- It does not ‘remember’ past exposure.
- It is genetically encoded in germ-line cells (sperm and eggs).
The last point requires some further discussion. Since the innate system is coded by germ cells, it is essentially set for life. However, microbes rapidly evolve and change characteristics, so the innate immune system alone cannot adapt to respond to these changes.
As mentioned, a key element in any immune system is the ability to distinguish ‘self’ from ‘non-self’. One of the main innate immune mechanisms for responding to non-self are toll-like receptors (TLRs) (Figure 42).
Figure 42. Toll-like receptors and their ligands
TLRS and Innate Immune Recognition
TLRs are one of the main (but not only) effectors of innate immune recognition, and are found on a variety of cells, but especially on macrophages and antigen-presenting cells. TLRs are integral to the innate immune response. Also called ‘pattern recognition receptors’, or PRRs, they can recognize broad molecular patterns associated with either pathogens (pathogen-associated molecular patterns, or PAMPS) or with molecules released by damaged cells (damage-associated molecular patterns, or DAMPS).
- TLR1 and TLR2 recognize lipoproteins which may be present in bacterial cell walls.
- TLR3 binds to double-stranded RNA (dsRNA) present in rotaviruses.
- TLR4 is activated by lipopolysaccharide (LPS) that is part of the cell wall of gram-negative bacteria such as Escherichia coli, Pseudomonas aeruginosa, and Neisseria gonococcus.
- TLR5 responds to flagellin, a protein found in bacterial flagella.
- TLR8 binds to single-stranded RNA (ssRNA) from viruses such as the common cold, influenza, and Ebola.
As discussed earlier, the first phase of an immune reaction, whether it is part of the innate or the acquired (adaptive) immune response, is recognition. The recognition phase of the innate immune response is far less specific compared the acquired immune response. The next phase, or response, that is triggered by TLR binding is multifaceted, but in brief involves activation of key cellular effectors such as antigen-presenting cells and other macrophages (Figure 43).
Figure 43: Innate immune effectors
Innate Immune Responses
Upon activation, several types of effector cells release effector molecules, some of which will be familiar from our discussion of the inflammatory response. A key cellular effector of innate immunity is the antigen-presenting cell (APC). There are several different types of APCs, including dendritic cells (which are resident in tissues) and macrophages (which can circulate among tissues). Dendritic cells that are specifically resident in skin are referred to as Langerhans cells, after Dr. Paul Langerhans. APCs and macrophages can recognize non-self via several different types of receptors, and participate in both the innate and acquired immune response. As part of its role in the innate immune system, APCs express TLRs on their surface. When TLRs are occupied by non-self proteins such as bacterial flagellin, one of the responses is to secrete a variety of cytokines, which in turn activate some of the cellular effectors of innate immunity. These include:
- Natural killer, or NK cells
- Monocytes
- Neutrophils
Natural killer cells can release factors that have cytolytic (cell-killing) effects against virus-infected and tumourous cells. Some of these factors include interferon-gamma (IFN-δ) and chemokines. Monocytes are the largest type of white blood cell which, upon activation, can leave the blood vascular system to function as a phagocytic cell. Neutrophils are the most numerous type of white blood cell, and their function is primarily to phagocytose pathogens such as bacteria. During bacterial infections, the numbers of neutrophils can increase dramatically, which is clinically referred to as an elevated white count.
Self-Study Question:
You will recall that we earlier introduced the mast cell as a major cellular effector of the inflammatory response. Here we see another overlap between the inflammatory response and innate immunity. Mast cells also possess TLRs, and thus can also be activated by non-self. When this occurs, histamines and chemotaxins are released, exerting vascular effects and recruiting other cells such as eosinophils. Characterized by their acidic (eosinophilic) granules, upon activation eosinophils release these granules, which can have cytotoxic effects on parasites. Eosinophils are now believed to function as APCs, and as we will learn later, eosinophils are also involved as initiators and perpetrators of asthma and allergic inflammation.
Self-Study Question:
What is the role of basophils in innate immunity?
Unit 3.4 The Acquired Immune Response
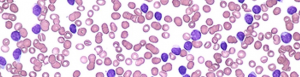
Acquired Immunity
The acquired immune response is not present at birth, and can require of period of 3–6 months postnatally before it is capable of mounting effective defences. Although there are several points of overlap between the innate and acquired immune responses, there are key differences. The following are key features of the acquired immune response:
- Specificity
- Memory
- Plasticity
Specificity refers to the ability to recognize specific non-self molecules, or antigens, including proteins and polysaccharides, rather than broader molecule patterns. The primary effectors of recognition are antibodies, or immunoglobulins, which belong to a larger ‘superfamily’ of immune globulins that we will discuss later.
Memory refers to the fact that the acquired immune system ‘remembers’ past exposure to antigens, and can respond more rapidly and to greater effect. This is reflected in the graph below, which depicts the kinetics of antibody production, as expressed by the log of antibody titre over time (Figure 44).
Figure 44: Immunoglobulin titres after repeated exposure to antigens
The relative amounts of two different antibodies, immunoglobulin G (IgG) and immunoglobulin M (IgM), are shown after exposure to an antigen (depicted by the arrows). Upon the first exposure, after a brief lag, there is an increase in both IgG and IgM. Upon re-exposure (the second arrow from left), there is a more rapid, larger, and sustained increase in IgG. This is indicative of components of the acquired immune system having ‘remembered’ prior exposure to that specific antigen.
Finally, plasticity refers to the ability of the acquired immune system to adapt to changes in antigens, and to develop tolerance to other antigens. This has implications for the way the immune system responds to disorders caused by viral infections, and to phenomena such as developing tolerance to allergens. For this and other reasons, the acquired immune system is often also referred to as the ‘adaptive’ immune system.
Antibodies
In basic structure, the generic antibody (Figure 45) consists of four polypeptides, including two ‘heavy’ chains, and two ‘light’ chains, joined by disulfide (S-S) bonds. Together, these chains form a ‘Y’ shape, at the tip of which (the two Fab fragments) is the antigen-binding site. Antigens can be relatively large; most antibodies bind to a specific region of the antigen called an epitope. The very tips of the Fab regions can have great differences in the amino acid sequence (recall that proteins and peptides are comprised of amino acids). This variability is what confers antibody-antigen specificity.
Figure 45: Structure of an antibody
The Fc fragment also has important functions: it interacts non-specifically with inflammation mediators and with receptors on B cells (Fc receptors).
There are five major classes of antibodies:
- IgA: found in secretions such as saliva
- IgG: approximately 75% of serum antibodies; multiple functions
- IgD: expressed in plasma membrane of B cells; activates B cells
- IgM: activates B cells; present as hexamers (units of 5) and pentamers (units of 6)
- IgE: essential for type I hypersensitivity reactions (discussed later); involved in response to parasites and allergies
Other members of the immunoglobulin superfamily include T cell receptors, major histocompatibility I and II receptors, coreceptors such as CD4 and CD8, NK receptors, and receptors for some cytokines. We will delve into some of these other members of the immunoglobulin superfamily later in this unit, although a key point to remember is that they are all involved in various ways in recognizing non-self.
Functions of Antibodies
Antibodies constitute one of the main effectors of the humoral immune response. As depicted in Figure 46, antibodies have multiple functions. However, these can be classified as having either one of the following effects:
- Neutralization: binding to pathogens or their secretions; blocking attachment of viruses or bacteria
a. Coating toxins
b. Clumping foreign agents - Enhanced killing
a. Activating the complement system
b. Inducing the release of oxidants
c. ‘Opsonizing’ or coating bacteria to make them easier to phagocytose
Figure 46: Functions of antibodies
At this point, let’s step back and introduce the principal cellular effectors of the acquired immune response: B cells, T cells, and APCs (recall that we’ve mentioned APCs in the previous section). We will start with B cells, as they are the only cells of the immune system which produce antibodies. Under the light microscope, B cells appear somewhat unremarkable: there is a large, central nucleus and scant cytoplasm with no visible granules (Figure 47).
Figure 47: Main features of B cells
B cells are so named because they were first identified in birds, where they are produced by an organ called the Bursa of Fabricus. Humans do not possess this organ, but the name has stuck. In humans, B cells and other white blood cells (leukocytes), as well as red blood cells (erythrocytes), are produced in red bone marrow. It is important to remember that a specific B cell produces a specific type of antibody. Since there are hundreds of thousands of different antigens, it follows that there are many different types of B cells. Each B cell has a B cell receptor (BCR), which is essentially a membrane-bound antibody, as well as major histocompatibility complex II receptors (MHC II) (more on these later). When a BCR binds to a specific antigen, that B cell is said to be primed. To become activated (which needs to occur before antibodies can be produced), a co-stimulus is required from another immune cell, the helper T cell (TH). If no co-stimulus is received, the B cell dies or is permanently inactivated. We will return to what happens when a B cell becomes activated; first, let’s introduce the main features and types of T cells.
T Cells
Under the microscope, it is not possible to distinguish T cells from B cells. However, T cells possess a unique receptor, the aptly-named T cell receptor (TCR) which, as part of the immunoglobulin superfamily, functions similarly to antibodies, binding to non-self antigens (Figure 48).
Figure 48: Main features of T cells
One important difference is that, unlike antibodies, TCRs can only bind to antigens that are ‘presented’ by other cells. Naïve T cells each have a unique TCR, plus a coreceptor, the CD4 protein. Other types of T cells have other coreceptors. For examples, cytotoxic T cells (TC) have the CD8 protein, and Tregs have the neuropilin coreceptor. These coreceptors are necessary for successful binding of the TCR to its antigen (Figure 49).
Figure 49: T cell sub-types
It is beyond our scope to go into great depth on the myriad functions of each of these cells; however, we can highlight some cardinal roles of some of these cells and their receptors:
- TCRs bind fragments of antigens presented via MHC I receptors on other cells.
- Antigen-presenting cells (APCs) always help activate T cells.
- B cells and T cells also interact for mutual activation.
In addition to a unique TCR, T cells also have other receptors:
- IL-1 receptors (IL-1r)
- Coreceptors
- Major histocompatibility complex (MHC) II
MHC II receptors are found on all ‘professional’ immune cells such as dendritic cells and B cells and, as part of the Ig superfamily, recognize specific antigens. Given that we have MHC II receptors, this begs the question: what are MHC I receptors? We will discuss these below, in the context of APCs.
Dendritic Cells
We introduced antigen-presenting cells (APCs) earlier while discussing the innate immune response. Dendritic cells and macrophages are both types of APCs. These multifunctional cells also participate in acquired immune response, and possess a variety of receptors for these purposes (Figure 50), including:
- MHC I receptors
- MHC II receptors
- TLR receptors
Figure 50: Antigen-presenting cells (dendritic cells)
APCs phagocytose intact antigens into phagolysosomes, after which, as their name implies, they present these antigen fragments to the TCR utilizing the MHC II receptor. At this point, it is convenient to describe the similarities and difference between MHC I and MHC II receptors. MHC I receptors display fragments of proteins produced within the cell displaying them (endogenous antigens, such as those produced by cells infected with viruses). These are found on all cells in the body, except for red blood cells. Antigens displayed by MHC I can be recognized by T cells carrying CD8 coreceptors (TC cells). MHC II differs from the MHC I receptor in that MHC II displays fragments of proteins produced outside the cell displaying them (exogenous antigens, such as those from bacteria). They are recognized by T cells carrying CD4 (TH cells) and expressed only by ‘professional’ antigen-presenting cells: B cells, macrophages, and dendritic cells.
As illustrated below, APCs can activate both cytotoxic (TC) and helper T (TH) cells by displaying endogenous and exogenous antigens via their MHC I and MHC II receptors, respectively. APCs activate both TC cells and TH cells (Figure 51).
Figure 51: Dendritic cell activation of TC and TH cells
Although APCs activate TC cells, the story doesn’t begin there. Initially, naïve TC cells live in lymphoid tissues, where they can be activated when the TCR/CD8 complex binds to antigens bound to MHC I receptors. Cytotoxic T cells bind to endogenous antigens displayed by MHC I receptors on certain ‘self’ cells (those which normally exist in the body), but which now display altered antigens, often as a result of damage or viral infection. One way to conceptualize this is that the APCs ‘confirm’ there is a problem, and only after doing so are TC cells fully activated to kill cells.
After being fully activated, some TC cells become memory cells, which can respond some time later to the antigen. Other TC cells immediately release cytotoxins, including perforins and granzymes (Figure 52). Perforins create holes in the target cell, after which granzymes enter the cell and activate caspases (recall that caspases are involved in apoptotic cell death). In this way, infected cells are eliminated from the body.
Figure 52: Mechanisms of TC-mediated cell killing
Let’s now turn back to B cell activation, and the roles of helper T cells. TH cells fully activate B cells by displaying exogenous antigen via their TCRs, utilizing their CD4 coreceptors (Figure 53).
Figure 53: Activation of B cells by TH cells
TH cells help to fully activate B cells, although B cells can also activate TH cells. This is because B cells can also act as antigen-presenting cells. Recall that antigen binding to the BCR only primes the B cell. Now, the B cell becomes fully activated by the TH. Once fully activated, the B cells now clones or exactly duplicates itself. Most clones become plasma cells, which secrete antibodies for that specific antigen. This now brings us back to one of the fundamental properties of the acquired immune response: memory. Recall that each specific antibody is produced by a specific B cell. Also recall that, after the initial exposure, the IgG titre rises, then falls. After a second exposure, the magnitude and duration of the antibody response is increased.
The reason? Once a specific B cell is activated, one of its first responses is to clone, or duplicate itself. While most clones become plasma cells, some also become memory cells, which are longer-lived, and remain in circulation (Figure 54).
Figure 54: Clonal expansion of B cells
This is referred to as clonal expansion. The implication of clonal expansion is that, after the initial exposure, there will be more memory B cells in circulation able to respond to that specific antigen (and thus produce a larger secondary response).
Finally, let’s summarize the ‘bigger picture’, illustrating the interactions between dendritic cells, TH cells, TC cells and B cells (Figure 55):
Figure 55: Overview of interactions between dendritic cells, T and B cells
Unit 3.5 Hypersensitivity, Autoimmunity, Immune Deficiencies
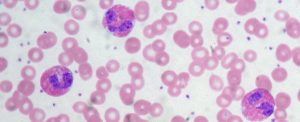
Hypersensitivity Reactions
We can now put into context some of the cellular and humoral effectors we’ve covered previously, and apply these to specific immune disorders. One common group of disorders involving the immune system are hypersensitivity reactions.
Hypersensitivity reactions occur when the normally-protective immune system responds with a maladaptive response which may cause harm. The allergic response is an immune reaction to an exogenous substance which, at first glance, seems normal. However, when an individual responds to an exogenous substance such as a protein which normally does not provoke a noticeable response in most individuals, it can be said to be a hypersensitivity. In the schema depicted below (Figure 56), we can see the typical ‘players’ or cells which respond to a foreign protein or allergen.
Figure 56: The allergic response
APCs (which can include dendritic cells, macrophages, and B cells themselves) activate TH cells by presenting an antigen such as a peanut protein via their respective MHC II receptors and TCR. Downstream, B cells become activated to become IgG- and IgE-producing plasma cells. IgE binds to its Fc receptor on mast cells, which then degranulate, releasing vasoactive factors that induce tissue hyperemia and swelling that can manifest itself as hives and soft tissue swelling. Thus, we’ve come full circle, wherein the allergic immune response essentially becomes an inflammatory condition – if severe enough, that is. If there is serious soft tissue swelling, bronchospasm, and loss of blood pressure, this allergic reaction becomes an anaphylactic, or Type I hypersensitivity. We typically classify hypersensitivity as either Types I, II, III, or IV, although in actuality, many hypersensitivity reactions overlap or combine elements of each other (Figure 57).
Figure 57: Hypersensitivity reactions I–IV
We can summarize the features and exemplars of hypersensitivity reactions by type as follows:
- Type I:
Referred to as ‘true allergies’, or anaphylaxis, this reaction is IgE-mediated, and occurs relatively rapidly (minutes).
eg: peanut allergies - Type II:
Also known as a cytotoxic reaction, this hypersensitivity reaction is mediated by antibodies (IgG or IgM) on the surface of cells or tissues. Cell lysis is mediated by the activation of complement proteins, which form a pore in the cell membrane with 3–6 hours.
eg: transfusion reactions - Type III:
This involves immune complex formation (antibody-antigen). These complexes form deposits in vascular tissues within kidneys, skin, and mucous membranes. Typically occurs within 3–6 hours.
eg: systemic lupus erythematosus, vasculitis - Type IV:
Also known as delayed (T-cell mediated) reaction, this typically takes several hours. Sensitized TH cells release cytokines that activate TC cells and macrophages attack on self proteins in skin, altered by reaction with sensitizers.- eg: poison ivy (and related plants)
- This reaction is the result of an oily secretion containing the molecule urushiol, which can penetrate outer skin. Urushiol covalently links to local proteins, which become antigenic, generating memory TC cells resident in skin. Subsequent exposure causes inflammation and tissue damage, usually within 2–4 days.
- eg: poison ivy (and related plants)
Autoimmune Reactions
These reactions are essentially attacks on normal self cells/molecules by the humoral and/or cellular arms of the acquired immune system. They typically cause inflammation and destruction of self cells, primarily via effects of antibodies, or the destruction of particular cell types by TC cells. Examples include:
- Type I diabetes: β cells in the pancreas
- Multiple sclerosis: myelin sheaths around neuron fibers (axons)
- Narcolepsy: small population of sleep-regulating neurons in the brain
What prevents autoimmunity? There are two theories:
- Pathogen-associated molecular patterns (PAMPS), which are molecular effectors of innate immunity, are one mechanism by which autoimmunity is presented.
APCs present foreign (non-self) proteins much more efficiently than they do self. During endocytosis, detection of PAMPs stimulates presentation of proteins taken in along with PAMPs. This leads to T cells being activated only if APCs provide co-stimulus (confirming that there is a ‘problem’). Since B cells need a co-stimulus from activated TH cells that recognize an antigen displayed by the B cell, lymphocytes that bind to an antigen, but do not receive a co-stimulus, will shut down (“anergy”). - ‘Private’ cellular compartments
Some tissues in the body are isolated from systemic circulation by tissue and/or cellular barriers
eg: synovial joints, brain tissues, germ cells
This theory posits that autoimmune diseases affecting these tissues are the result of breakdown of barriers and the exposure of these tissue antigens to immune cells.
Self-Study Question:
Immune Deficiencies
Approximately 25% of immune disorders are the result of autoimmunity. The remaining immune disorders are primarily the result of hypofunctional immune mechanisms, which can present as:
- Frequent infections
- Unusual infections
- Cancers
The cause of most immune deficiencies may be classified as either primary or secondary. Primary immune deficiencies are usually the product of a single gene defect that is not inherited, and symptoms can be early- or late-onset. Some examples include:
- Wiskott-Aldrich (B & T cell defect): signs include eczema, thrombocytopenia
- DiGeorge syndrome (T cell defect): signs include facial anomalies, low serum Ca++, cardiac defects
- Severe combined immune deficiency (SCID): many forms, the most common of which is X-linked SCID. The principle cause is a defect in the IL-2 receptor, which is needed for T and NK cell development.
Secondary immune deficiencies are more common, and are not defined as genetic. Typical causes include:
- Drugs such as prednisone and cyclosporine
- Viruses may also cause immune deficiencies; perhaps the best-known is acquired immunodeficiency syndrome (AIDS), which is caused by infection with the human immunodeficiency virus (HIV). HIV preferentially attacks TH cells (which have the CD4 receptor).
Self-Study Question:
SUMMARY
The inflammatory response and the innate and acquired immune systems collectively defend against foreign pathogens and other ‘non-self’ entities, including virally-infected cells and cancerous cells. Many disease states are the result of inflammatory and/or immune disorders, or induce elements of each. While the immune response in particular is complex and incompletely understood, there are fundamental differences between innate and acquired (adaptive) immunity, as well some of the common cellular and molecular effectors of each. We have also discussed the differences between primary and secondary immune disorders. Rather than focus on the myriad ‘trees’ that comprise the details of the immune responses, instead look at the ‘forest’, or overall concepts, as highlighted in the self-study questions and practice problems.
lipopolysacchride; large molecule consisting of a lipid, a polysaccharide, and an 'O-antigen'. Component of gram-negative bacterial cell walls
Tumor Necrosis Factor alpha, or cachexin, a cell signalling protein involved in systemic inflammation and the acute phase reaction
tail region of an antibody that interacts with cell surface receptors
class of transmembrane proteins that recognize structurally-conserved molecules from microbes' found on immune sentinel cells such as dendritic cells or macrophages
a class of G-protein-coupled receptors that bind histamine; four types have been identified
small signalling proteins which induce chemotaxis or cell movement
factors secreted by cells (particularly but not exclusively immune cells) which affect other cells; includes interferons and interleukins
small molecules conserved in microbes; activate innate immune responses
danger or alarm signals that can initiate an inflammatory response; may be nuclear or cytoplasmic proteins released by damaged cells
any cell that assists in the immune responses by displaying antigens in association with their MHC II receptor; T cells may then recognize these antigens via their T cell receptors
a protein that is a principal component of bacterial flagella
a way of expressing concentration; corresponds to a dilution factor that still yields a positive reading
coating and making a molecule more susceptible to phagocytosis
cell surface proteins that bind to non-self proteins, making them accessible to binding with other receptors
production of identical daughter cells from a single cell
absence of a normal immune response to a particular antigen or allergen