Addressing Physical Accessibility Issues in Science Laboratories
Robert Szostak
Introduction
Science has been at the forefront of humanity’s development, leading to discoveries that have contributed to the betterment of the lives of many. We better understand our world through the cumulative efforts of scientists such as Charles Darwin and Isaac Newton. For centuries, women have been excluded from participating in this process due to their sex, resulting in the domination of ideas from one group and preventing different experiences and perspectives from being synthesized with scientific academia (Pitman, 2023). Various factors, such as societal expectations and systemic barriers, have posed a problem (Pitman, 2023); however, the representation of women in science has been increasing with time (Entradas, 2023). This issue has been discussed, but the same line of thinking and problems can be connected to those dealing with physical disabilities in science.
Laboratory work is integral to scientific education (Hofstein & Lunetta, 2004); it is often utilized in education to provide hands-on experience. Nevertheless, there are accessibility barriers for students, such as inaccessible workspaces and equipment (Fiss et al., 2023). When faced with these barriers, pursuing a career in science can become daunting and lead to systemic exclusion. Hence, the purpose of this analysis is to examine these barriers and look at potential solutions that can be implemented for those with physical disabilities.
Barriers for Those with Physical Disabilities in a Laboratory
Despite being an integral part of the science curriculum, laboratories possess a host of systemic barriers that can exclude those with physical disabilities from succeeding in this field. Those with physical disabilities may have conditions restricting mobility, hindering them from participating in activities (Goodwin & Watkinson, 2000). Jeannis et al. (2019) outline two significant barriers preventing students from fully engaging within a laboratory. The first pertains to physical accessibility, preventing students from engaging in an assigned task. Entering the building or laboratory room is a common barrier. Within the lab, aisles between benches are too narrow, stools block pathways, workspaces are cramped, or items are out of reach. The second barrier relates to task execution, with half of the participants limited to passive roles; watching a lab partner complete the task, or only taking notes.
Inclusive environments that promote collaboration and meaningful interactions boost students’ learning (Santiago-Garabieta et al., 2023). Delegating students to take notes or make observations inhibits them from becoming meaningful participants. Accessible microscopes were an issue for some students (Jeannis et al., 2019). If students cannot gain essential skills, it creates an additional challenge during their scientific career when they graduate. Additionally, it may be inferred that the work environment can be a safety hazard, ranging from accidental chemical spills to fires.
Figure 1
Front of a Building at the University of Manitoba
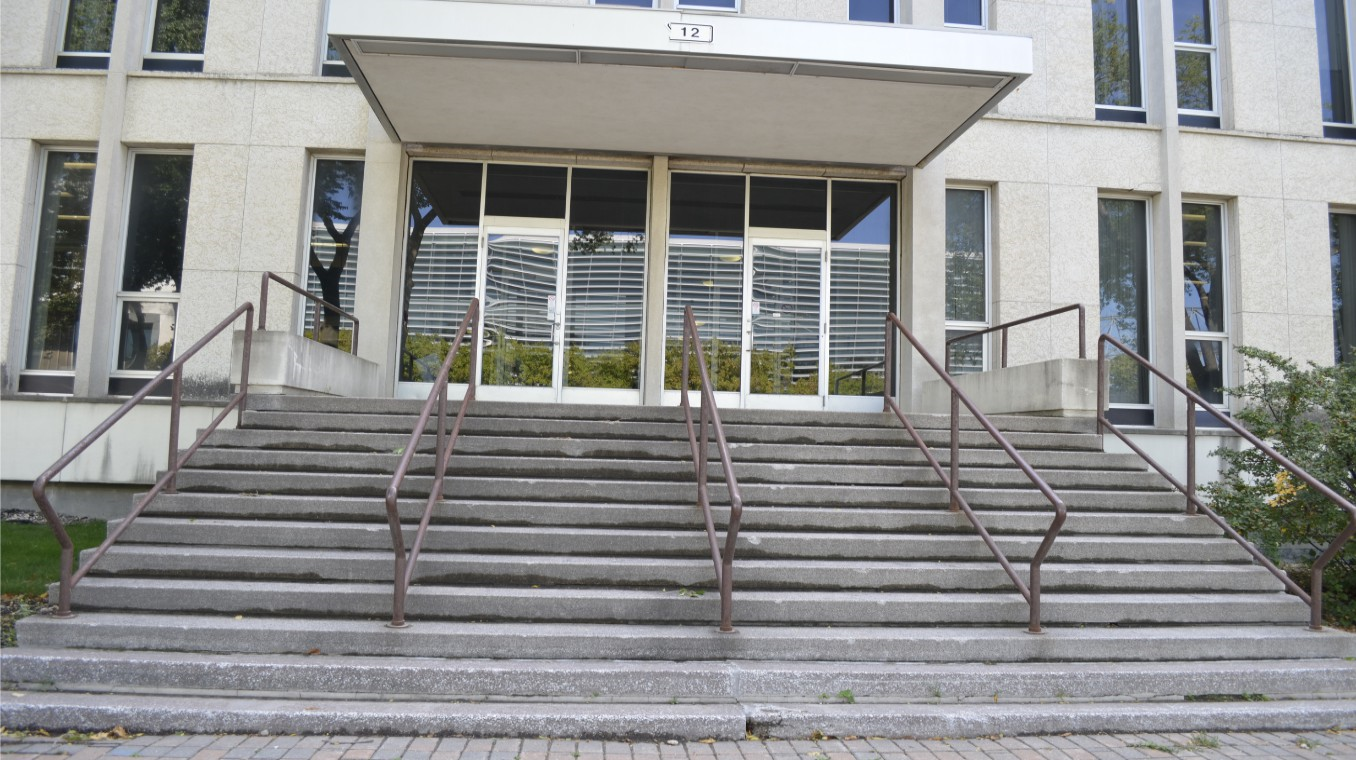
Although some steps have been implemented in institutions, it still needs to be improved. Bergen (2019) looked at accessibility for the University of Manitoba (Figure 1); there has been some positive reception with additions such as ramps; however, it is still a negative experience for students. She found there are still physical barriers, such as elevators on top of short stairwells or the need for more power door openers. The Accessibility for Ontarians with Disabilities Act (AODA) mandates that the province be accessible by 2025 or face fines (Jacobs, 2016). Therefore, it is important to consider approaches that can help meet these standards.
Current Accessibility Practices
To help address these barriers, laboratories have been modified to make them more accessible. Hilliard et al. (2013) review modifications that adhere to accessibility guidelines for lab retrofitting. Some key areas were adjustable height workbenches, lowered sink counters, lowered fume hoods, and a lower chain for emergency shower stations for users with wheelchairs (Figure 2). While an improvement, there are still limitations to these adjustments. Depending on the individual, impairments can vary between difficulty with movement and being unable to utilize wheelchairs due to upper-body mobility issues (Iezzoni et al., 2000). There is also still a problem relating to implementation.
Retrofitting labs can be a significant undertaking. Some changes include changing cabinet heights, adding automatic door openers, replacing old wooden benchtops, or adding adjustable seating (Egambaram et al., 2022). Hence, retrofitting buildings to make them more functional comes with a high cost (Solanki et al., 2022). In higher education, funding has been static despite the increasingly complex needs of students, resulting in higher costs (Lanthier et al., 2023). These expenditures and decreased funding can become prohibitive, leading to inconsistent accessibility standards across educational institutions and lower standards. I have observed reactive measures such as buying a height-adjustable desk when needed rather than being proactive and implementing it beforehand in a newly renovated lab. Because of a lack of resources, students continue to encounter labs that are not fully accessible, with educators unsure how to meet legal requirements for students while balancing cost, health, and safety in this legal gray zone (Prema & Dhand, 2019). Therefore, it is crucial to consider instructional design strategies and learning resources that could be utilized to make labs more accessible, which would make the best use of funding and the limited resources available to educational institutions.
Figure 2
Examples of Accessible Retrofitting in a Lab
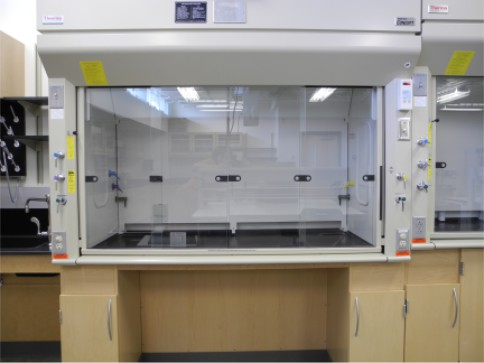
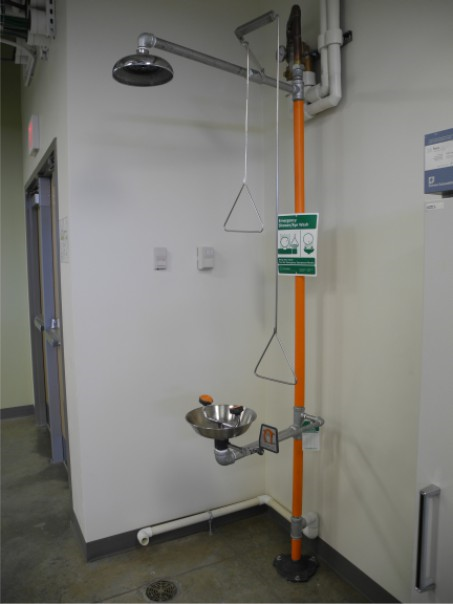
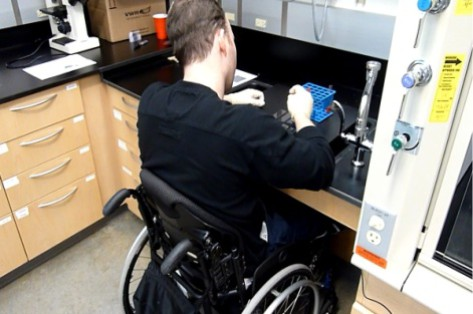
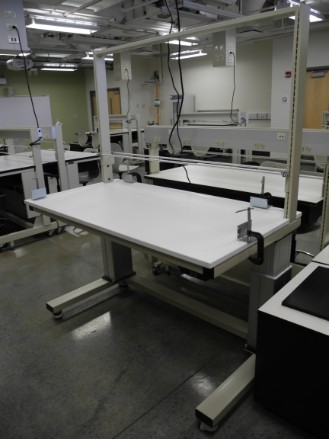
Note. Various accessible features can be used in a lab: (a,c,d) lowered work environments, (b) extended safety shower chains that can be pulled. From “Designing Beyond the ADA—Creating an Accessible Research Laboratory for Students and Scientists with Physical Disabilities,” by L. Hilliard, P. Dunston, J. McGlothlin, & B. S. Duerstock, 2013, presented at the RESNA Conference.
Alternative Practices to Address Barriers
An instructional design strategy that could mitigate these barriers is the universal design for learning (UDL). This framework leads educators to make learning more inclusive by providing multiple means of representation, expression, and engagement (Ralabate, 2011). Each one of these areas can be expanded upon further to address lab inaccessibility. Representation looks at students’ learning, providing options for perception (Kohnke et al., 2022). Virtual simulations have been used to simulate lab practices, but were found not to be as hands-on and were not effective in giving experience to working with others (Ma & Nickerson, 2006); considering the purpose of labs to teach these skills, simulations can be reviewed further with the advent of virtual reality and artificial intelligence. Expression is how learning is done, providing options for physical action (Kohnke et al., 2022).
Traditional microscopes can challenge those with mobility impairments (Jeannis, 2018). Instead, institutions can provide digital microscopes, which can be controlled via a computer and show what is being viewed on the screen (Hartati et al., 2011). Engagement looks at the why of learning, which provides options for effort (Kohnke et al., 2022).
Depending on the situation, students could perform roles that best suit their physical ability. However, it is crucial to be mindful that they are not put in passive roles such as only recording observations or calculations (Jeannis et al., 2019).
Expanding further on previously mentioned virtual simulations, this can provide an accessible alternative to a traditional setting concerning virtual reality (VR) and artificial intelligence (AI). As illustrated by Figure 3, VR is a media system that provides interactive, three-dimensional virtual environments, replacing sensory input with computer-generated visuals and sound (Huang et al., 2023). AI is where a machine can communicate, reason, and operate independently, like a human (Du-Harpur et al., 2020). Considering the intersectionality between these two technologies, VR lab simulations using AI could be an area to consider further to train students in laboratory practices. Kaviyaraj and Uma (2021) found that VR can provide an alternative way to provide real-world experience, such as in a physical setting for laboratory work. This technology can be implemented within schools with limited laboratory funding to provide a good learning experience (Bogusevschi et al., 2020).
Figure 3
Simulated Virtual Reality Classroom
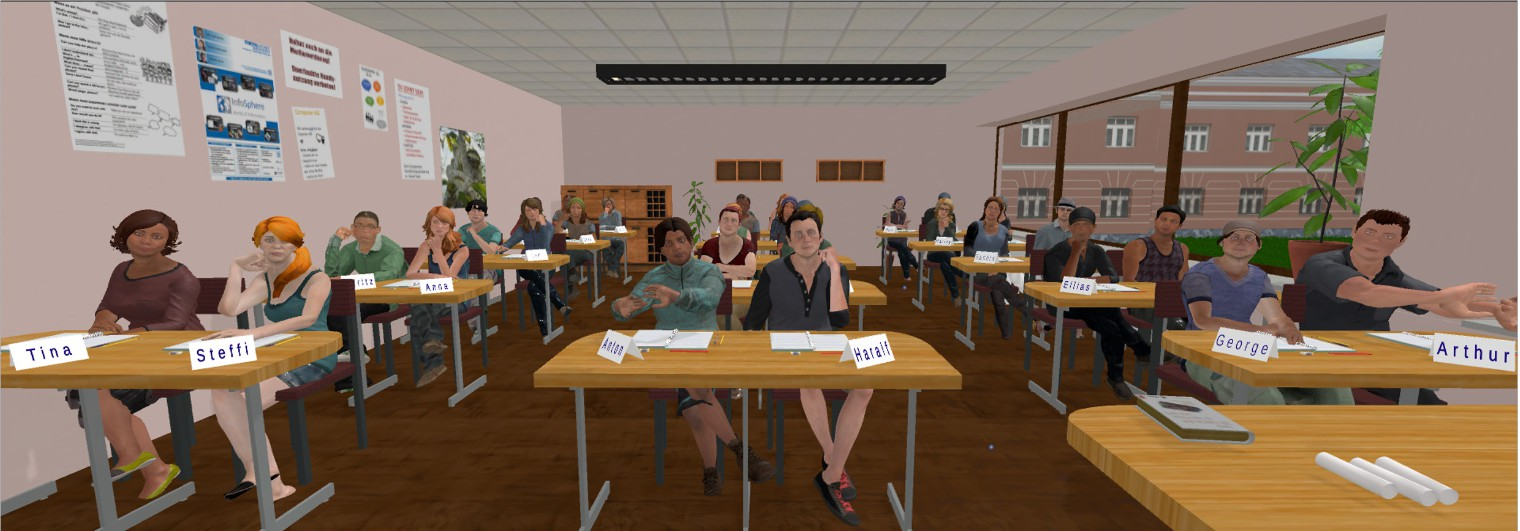
However, VR could be limited to what is programmed within a developed piece of lab software. AI could provide this change by implementing AI lab partners and procedural generation, creating a more immersive learning environment. Although AI is still developing, the Generative Pre-trained Transformer 4 (GPT-4) large language model (LLM) has been used as an instructional support in a VR laboratory pertaining to thermal fluids using the Unity Game Engine, providing reliable instruction and helping to troubleshoot problems much like a real-life lab assistant (Ayre et al., 2023). Simulating lab spills and communicating with partners provide a dynamic that is lacking in previous iterations of virtual simulations. Either way, virtual simulations have also been found to provide more time and a safer environment for students, with the main criticism being the lack of building collaborative team skills (Shanab et al., 2012).
Considering these two approaches, UDL and VR with AI can be synthesized to provide a holistic learning experience for students with physical impairments. It provides a practical learning experience (Huang et al., 2023) that utilizes an accessible representation method via the construction of a three-dimensional space. It can be more engaging than traditional learning methods (Huang et al., 2023), providing sustained interest for learners.
Lastly, it can be a helpful tool to provide instant feedback for students (Onesi-Ozigagun et al., 2024), making it an effective assessment tool for evaluating lab practices. This approach provides a cheaper yet effective alternative (Bogusevschi et al., 2020), as retrofitting buildings can be expensive (Solanki et al., 2022). Considering safety, it also minimizes risks that could be prevalent in a chemistry setting where it can be easy for a hazardous spill to land on someone seated, making it difficult for someone to make it to an eye-wash station or shower when, considering the previously mentioned barriers of cluttered work-spaces and narrow aisles. Although some limitations should be considered, as Huang et al. (2023) found, this learning method had a higher extraneous load than traditional methods; however, this could have been attributed to insufficient training and participants having to grapple with unfamiliar controls while trying to learn. Considering these points, it does not need to apply only to those with disabilities. With proper investments from educational institutions, this approach could provide an alternative way to train students in science labs, depending on what is being taught or assessed.
Accessible Resources
Considering the above mentioned, there are some resources that educators could refer to to create an accessible lab environment. Depending on the area being taught, traditional simulations are usually point-and-click within individual learning modules (Alvarez, 2021). There is a wide abundance of simulations that could be utilized:
- Labster (Alvarez, 2021; Labster, 2024)
- BioInteractive (Alvarez, 2021; BioInteractive.org, n.d.)
- Michigan State University (Alvarez, 2021)
- pHET (Taibu et al., 2021; University of Colorado, 2024)
These resources are available at a low cost or are entirely free on the internet (Alvarez, 2021). A wide variety of digital microscope platforms can be selected, such as the DG-X and ITRC-001 (Hartati et al., 2011). There are also more holistic platforms that aim for users to use light microscopy without any assistance at all, such as the AccessScope (Figure 4); this accessible interface provides remote control, an accessible graphic user interface, slide auto-loaders, a digital camera, an automated research microscope, and a motorized stage (Mansoor et al., 2010). In terms of attitudinal barriers with instructors in the laboratory, it was brought up that often, those with physical disabilities are put into passive roles; instructors must make them active participants by modifying the curriculum and procedures by seeking the help of experts using campus disabilities resource centers or in direct connection with the student (Jeannis et al., 2019).
Figure 4
AccessScope Light Microscope
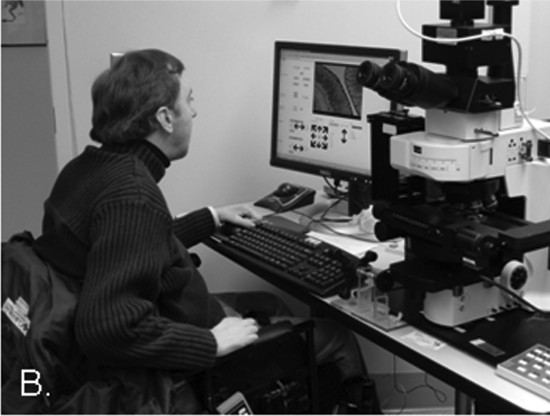
VR and AI are relatively new areas to consider, but VR laboratories have been used prior. Labster is a commercial system providing lab activities that integrate well with known learning management systems (Höhner et al., 2022). It is available as a subscription service (Wright, 2020). This type of software is more hands-on and engaging than traditional lab simulations and is well-accepted (Shanab et al., 2012). Headsets purchased to be used in a lab could include the PlayStation VR, Oculus Rift, HTC Vive, or the Diger (Çankaya, 2019). A computer will also be required to pair with VR headsets, as the hardware is connected. Different AI models are available for further exploration with their recent rise, such as ChatGPT, Bard, or Bing’s AI (Crawford et al., 2023).
Future Accessibility in Science Laboratories
This chapter highlights the accessibility barriers that are still present today for those with physical disabilities within a science laboratory. Although some solutions have been utilized, such as retrofitting entrances with elevators or ramps, gaps still prevent labs from being fully accessible. These gaps pertain to physical layout and instructor attitudes, whether in cramped and inaccessible lab workplaces or delegating students to passive roles. Retrofitting laboratories can be expensive due to static funding, and it takes time. A medium could be implemented utilizing UDL and new digital technological resources such as digital microscopes or VR. VR has been proven to be as effective as laboratory settings in teaching skills, with the additional benefits of not rushing students and working in a safer environment. Augmenting this technology with AI can further enhance the immersive experience of simulations with generative lab settings.
Educators have a vast catalog of resources regarding accessible technology and digital resources. Ensuring accessibility is essential to meet AODA standards and allow for greater inclusion within the scientific community. Creating positive learning spaces where students can participate safely and actively within labs is crucial, as it promotes their integration and efficacy within science. These resources are not just viable for those with physical disabilities but, if integrated, could provide a framework where all students can benefit, similar to other accessible technologies such as screen readers or voice assistants.
References
Alvarez, K. S. (2021). Using virtual simulations in online laboratory instruction and active learning exercises as a response to instructional challenges during covid-19. Journal of Microbiology & Biology Education, 22(1), 10–1128.
Ayre, D., Dougherty, C., & Zhao, Y. (2023). Implementation of an artificial intelligence (ai) instructional support system in a virtual reality (VR) thermal-fluids laboratory. ASME International Mechanical Engineering Congress and Exposition, 87653, V008T09A029.
Bergen, K. (2019). Planning and designing accessible public spaces in Canadian universities: A case study of the University of Manitoba. [Master’s thesis]. University of Manitoba. http://hdl.handle.net/1993/34219
BioInteractive.org (n.d.). Empowering Educators. Inspiring Students. https://www.biointeractive.org/
Bogusevschi, D., Muntean, C., & Muntean, G.-M. (2020). Teaching and learning physics using 3d virtual learning environment: A case study of combined virtual reality and virtual laboratory in secondary school. Journal of Computers in Mathematics and Science Teaching, 39(1), 5–18.
Çankaya, S. (2019). Use of VR headsets in education: A systematic review study. Journal of Educational Technology and Online Learning, 2(1), 74–88.
Crawford, J., Cowling, M., Ashton-Hay, S., Kelder, J.-A., Middleton, R., & Wilson, G. S. (2023). Artificial intelligence and authorship editor policy: ChatGPT, Bard, Bing, AI, and beyond. Journal of University Teaching & Learning Practice, 20(5), 1.
Du-Harpur, X., Watt, F., Luscombe, N., & Lynch, M. (2020). What is AI? applications of artificial intelligence to dermatology. British Journal of Dermatology, 183(3), 423–430.
Egambaram, O., Hilton, K., Leigh, J., Richardson, R., Sarju, J., Slater, A., & Turner, B. (2022). The future of laboratory chemistry learning and teaching must be accessible. Journal of chemical education, 99(12), 3814–3821.
Entradas, M. (2023). Women in science: Rising numbers but an eternal glass ceiling. Cultures of Science, 6(1), 23–33.
Fiss, B. G., D’Alton, L., & Noah, N. M. (2023). Chemistry is inaccessible: How to reduce barriers for disabled scientists. Nature, 623(7989), 913–915.
Goodwin, D. L., & Watkinson, E. J. (2000). Inclusive physical education from the perspective of students with physical disabilities. Adapted physical activity quarterly, 17(2), 144–160.
Hartati, S., Harjoko, A., & Supardi, T. W. (2011). The digital microscope and its image processing utility. TELKOMNIKA (Telecommunication Computing Electronics and Control), 9(3), 565–574.
Hilliard, L., Dunston, P., McGlothlin, J., & Duerstock, B. S. (2013). Designing beyond the ADA: Creating an accessible research laboratory for students and scientists with physical disabilities. RESNA Conference.
Hofstein, A., & Lunetta, V. N. (2004). The laboratory in science education: Foundations for the twenty-first century. Science education, 88(1), 28–54.
Höhner, N., Mints, M. O., & Rodewald, J. (2022). Integrating virtual and augmented reality applications into a connected laboratory environment. International Conference on Remote Engineering and Virtual Instrumentation, 585–594.
Huang, Y., Richter, E., Kleickmann, T., & Richter, D. (2023). Comparing video and virtual reality as tools for fostering interest and self-efficacy in classroom management: Results of a pre-registered experiment. British Journal of Educational Technology, 54(2), 467–488.
Iezzoni, L. I., McCarthy, E. P., Davis, R. B., & Siebens, H. (2000). Mobility impairments and use of screening and preventive services. American Journal of Public Health, 90(6), 955.
Jacobs, L. (2016). ’Humanizing’ disability law: Citizen participation in the development of accessibility regulations in Canada. Disability Law: Citizen Participation in the Development of Accessibility Regulations in Canada (May 31, 2016). Revue Internationale des Gouvernements Ouverts, 93–120.
Jeannis, H., Goldberg, M., Seelman, K., Schmeler, M., & Cooper, R. (2019). Barriers and facilitators to students with physical disabilities’ participation in academic laboratory spaces. Disability and Rehabilitation: Assistive Technology.
Jeannis, H. (2018). Barriers and facilitators in training students with physical disabilities in science and engineering laboratories [Doctoral dissertation, University of Pittsburgh].
Kaviyaraj, R., & Uma, M. (2021). A survey on the future of augmented reality with AI in education. 2021 International Conference on Artificial Intelligence and Smart Systems (ICAIS), 47–52.
Kohnke, S., Patterson, M. S., & Moehlmann, R. (2022). Udl solutions for common science barriers. The Science teacher (National Science Teachers Association), 89(6), 29–33.
Labster (2024). Inspire Students with Immersive STEM Learning. https://www.labster.com/
Lanthier, S., Tishcoff, R., Gordon, S., & Colyar, J. (2023). Accessibility services at Ontario colleges and universities: Trends, challenges, and recommendations for government funding strategies. Higher Education Quality Council of Ontario. https://heqco.ca/wp-content/uploads/2023/11/Accessibility-Services-at-Ontario-Colleges-and-Universities-FINAL-English.pdf
Ma, J., & Nickerson, J. V. (2006). Hands-on, simulated, and remote laboratories: A comparative literature review. ACM Computing Surveys (CSUR), 38(3), 7–es.
Mansoor, A., Ahmed, W. M., Samarapungavan, A., Cirillo, J., Schwarte, D., Robinson, J. P., & Duerstock, B. S. (2010). Accessscope project: Accessible light microscope for users with upper limb mobility or visual impairments. Disability and Rehabilitation: Assistive Technology, 5(2), 143–152.
Onesi-Ozigagun, O., Ololade, Y. J., Eyo-Udo, N. L., & Ogundipe, D. O. (2024). Revolutionizing education through AI: A comprehensive review of enhancing learning experiences. International Journal of Applied Research in Social Sciences, 6(4), 589–607.
Pitman, K. (2023). How centuries of sexism excluded women from science – and how to redress the balance. Nature (London), 619 (7969), 243–244.
Prema, D., & Dhand, R. (2019). Inclusion and accessibility in stem education: Navigating the duty to accommodate and disability rights. Canadian Journal of Disability Studies, 8(3), 121–141.
Ralabate, P. K. (2011). Universal design for learning: Meeting the needs of all students. The ASHA Leader, 16 (10), 14–17.
Santiago-Garabieta, M., Zubiri-Esnaola, H., García-Carrión, R., & Gairal-Casadó, R. (2023). Inclusivity, friendship and language learning: Boosting collaboration in interactive groups. Educational Research, 65(2), 189–203.\
Shanab, S. A., Odeh, S., Hodrob, R., & Anabtawi, M. (2012). Augmented reality internet labs versus hands-on and virtual labs: A comparative study. Proceedings of 2012 international conference on interactive mobile and computer aided learning (IMCL), 17–21.
Solanki, S. K., Rastogi, R., & Paul, V. K. (2022). Cost analysis of functional retrofitting measures in buildings. Journal of the Institution of Engineers (India). Series A, Civil, architectural, environmental and agricultural Engineering, 103(3), 725–732.
Taibu, R., Mataka, L., & Shekoyan, V. (2021). Using phet simulations to improve scientific skills and attitudes of community college students.
University of Colorado (2024). PhET: Interactive Simulations for Science and Math. https://phet.colorado.edu/
Wright, D. (2020). The online teacher: Conducting science labs in a virtual world. Science Scope, 44(2), 10–15.