Levels of Organization
8 Transport Across the Plasma Membrane
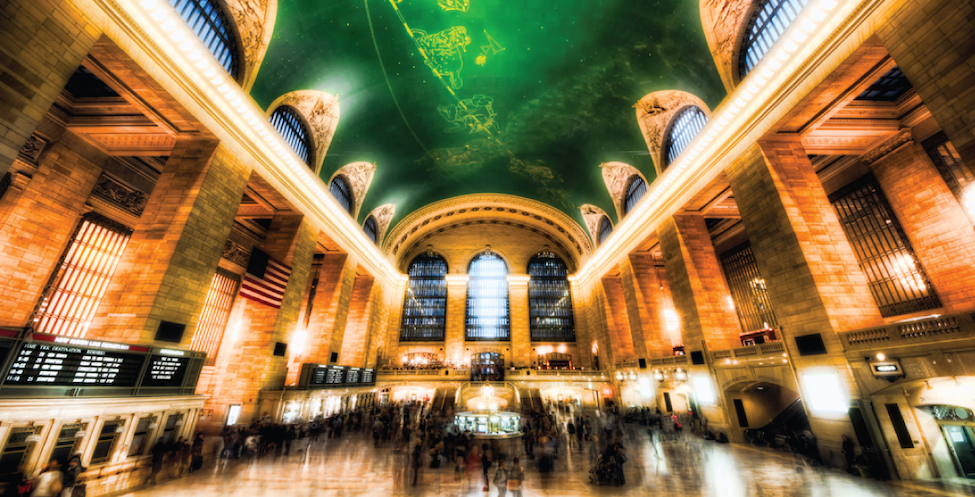
Despite its seeming hustle and bustle, Grand Central Station functions with a high level of organization: People and objects move from one location to another, cross boundaries or are contained within them, and create a constant flow as part of a larger activity. Analogously, a plasma membrane’s functions involve movement within the cell and across boundaries’ activities.
Structure of the Plasma Membrane
Learning Objective
There are many functions of the plasma membrane, also called the cell membrane, but the most basic are that it defines the cell, outlines its borders, and determines the nature of its interaction with its environment. It allows cells to exclude some substances, take in others, and excrete still others in controlled quantities. The plasma membrane must be very flexible to allow certain cells, such as red and white blood cells, to change shape as they pass through narrow capillaries. These are the more obvious plasma membrane functions. In addition, the plasma membrane’s surface carries markers that allow cells to recognize one another, which is vital for tissue and organ formation during early development and later plays a role in the immune response’s “self” versus “non-self” distinction.
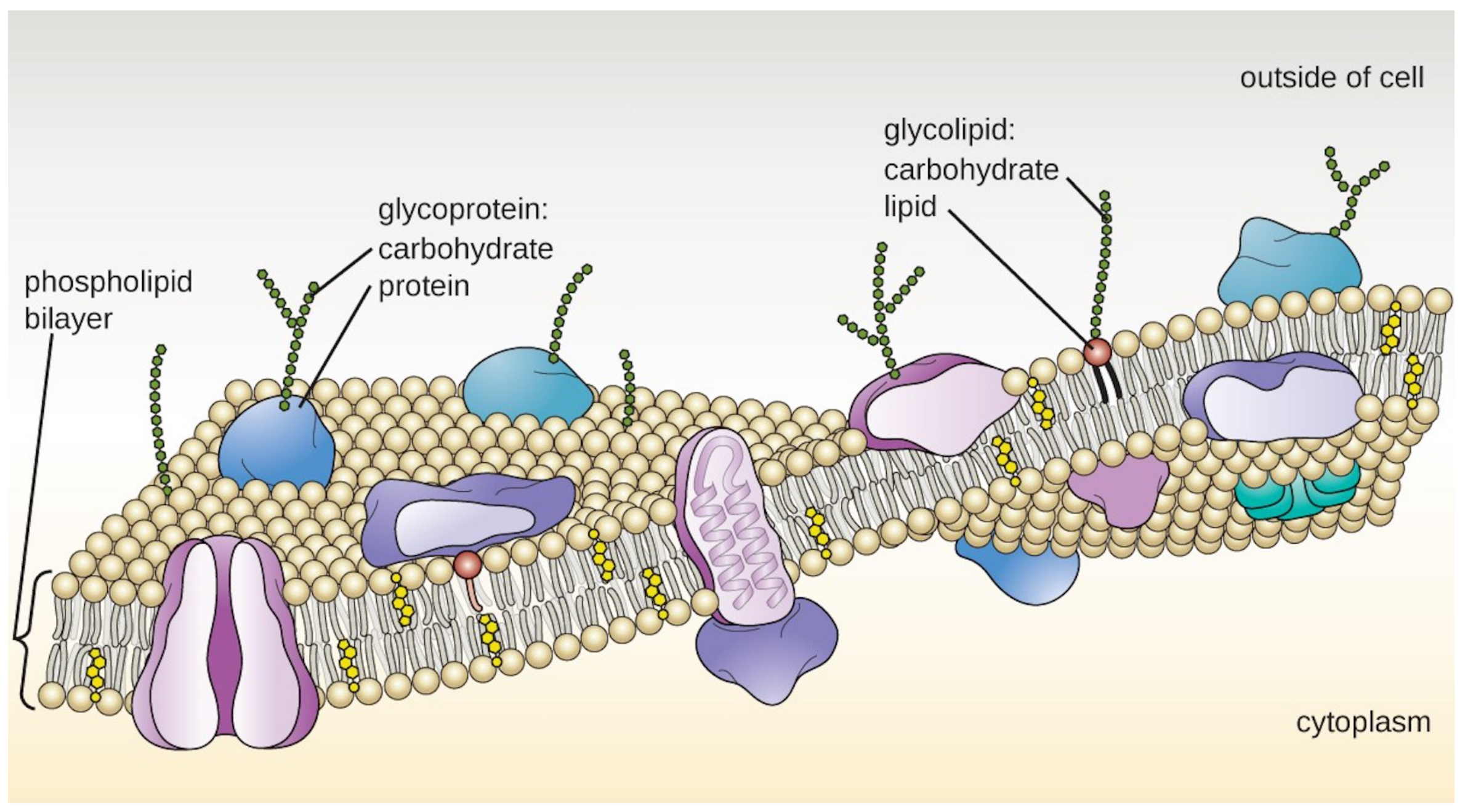
Among the most sophisticated plasma membrane functions is the ability of complex, integral proteins called receptors to transmit signals. These proteins act both as extracellular input receivers and as intracellular processing activators. These membrane receptors provide extracellular attachment sites for effectors like hormones and growth factors, and they activate intracellular response cascades when their effectors are bound. Occasionally, viruses hijack receptors (SARS-CoV2, the coronavirus that causes Covid-19 is one example) that use them to gain entry into cells. At times, the genes encoding receptors become mutated, causing the signal transduction process to malfunction with disastrous consequences.
In the previous chapter, you learned that the phospholipid bilayer is the main fabric of the membrane. The bilayer’s structure causes the membrane to be semi-permeable. Phospholipid molecules are amphiphilic (amphipathic), which means they contain both a nonpolar and polar region. Recall that phospholipids have a polar head which includes a charged phosphate group) with two nonpolar hydrophobic fatty acid tails. The tails of different phospholipids face each other in the core of the membrane, while the polar heads face either the exterior or interior of the cell.
Having the polar heads oriented toward the external and internal sides of the membrane allows them to interact with the polar water molecules on each side. The hydrophobic core blocks the diffusion of hydrophilic ions and polar molecules. Small hydrophobic molecules and gases can dissolve in the membrane’s core and easily cross it. Other molecules require proteins to transport them across the membrane. Proteins determine most of the membrane’s specific functions. The plasma membrane and the membranes of the various organelles each have unique collections of proteins. For example, more than 50 kinds of proteins have been found in the plasma membrane of red blood cells alone.
Importance of Membrane Structure
What is important about the structure of a phospholipid membrane? First, it is fluid. This allows cells to change shape, permitting growth and movement. The membrane’s fluidity is regulated by the types of phospholipids and the presence of cholesterol. Second, the phospholipid membrane is selectively permeable. The ability of a molecule to pass through the membrane depends on its polarity and, to some extent, its size. Selectively permeable membranes allow cells to keep the chemistry of the cytoplasm different from that of the external environment. It also allows them to maintain chemically unique conditions inside their organelles.
The fluidity of Cell Membranes
The cell membrane is not a static structure. It is a dynamic structure that allows for the lateral movement of phospholipids and proteins. Fluidity is a term used to describe the ease of movement of molecules in the membrane, an important characteristic of cell function. Fluidity depends on the temperature (increased temperatures increase fluidity, and decreased temperatures decrease fluidity) and the concentrations of saturated and unsaturated fatty acids. Saturated fatty acids make the membrane less fluid, while unsaturated fatty acids make it more fluid. The correct ratio of saturated to unsaturated fatty acids keeps the membrane fluid at any temperature conducive to life. In animal cells, cholesterol helps to prevent the packing of fatty acid tails and thus lowers the requirement of unsaturated fatty acids. This helps maintain the fluid nature of the cell membrane without it becoming too liquid at body temperature.
Learn By Doing 7.1
Describe the functions of the plasma membrane.
Membranes are described in all of the following ways except
- semipermeable barriers
- are fluid structures dependent on cholesterol and saturated fat composition
- will allow the transport of ions through the lipid layer
- uniform
- lipid bilayer
Plasma Membrane Proteins
Membranes also contain proteins, which carry out many of the functions of the membrane. Some functions of membrane proteins are:
- Junctions: Membrane proteins help cells bind to each other to form tissues (intercellular joinings). One example of this is skin cells, which must form a tight, waterproof connection between the cells to maintain the integrity of the skin as an organ.
- Enzymes: Membrane proteins can be enzymes involved in the chemical reactions of metabolism. These processes allow the cell to grow, obtain energy, and eliminate waste.
- Transport: Because the plasma membrane provides a barrier to the movement of many molecules, the cell needs a way to transport larger materials and hydrophilic molecules into and out of the cell.
- Recognition: Carbohydrate groups attached to proteins are used in cell-cell recognition, as described below.
- Anchorage: Other membrane proteins also bind to structural molecules inside and outside the cell to maintain cellular structure. This is referred to as anchorage or attachment.
- Transduction: Because the plasma membrane is the border of the cell, this is where the cell communicates with its environment. Membrane proteins can receive signals from outside the cell and begin a chain of events that cause the cell to respond to these signals. This process is called signal transduction.
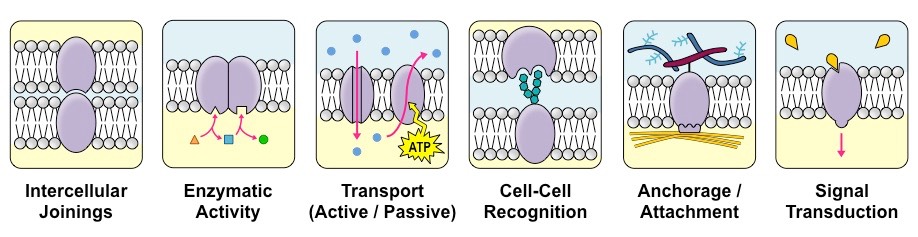
Membrane proteins are classified into two major categories: integral and peripheral proteins. Integral membrane proteins are those embedded in the lipid bilayer and generally characterized by their solubility in nonpolar, hydrophobic solvents. Transmembrane proteins are integral proteins with hydrophobic regions that completely span the membrane’s hydrophobic interior. The parts of the protein exposed to the interior and exterior of the cell are hydrophilic. Integral proteins can serve as pores that selectively allow ions, nutrients, and wastes into or out of the cell. They can also transmit signals across the membrane.
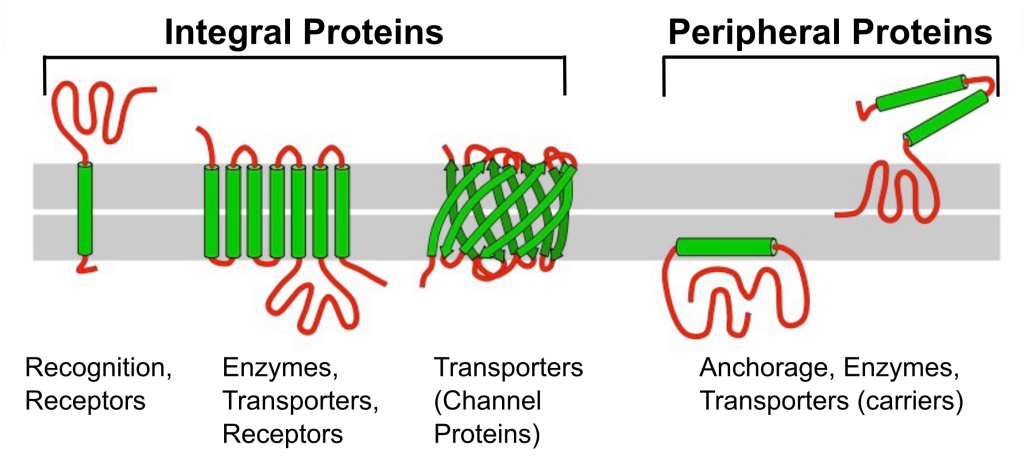
Unlike integral proteins that span the membrane, peripheral proteins reside on only one side of the membrane and are often attached to integral proteins. Some peripheral proteins serve as anchor points for the cytoskeleton or extracellular fibers. Proteins are much larger than lipids and move more slowly than phospholipids in the membrane. Some move in a seemingly directed manner, while others drift. Some are glycoproteins which have a carbohydrate group attached to the protein. These are on the outside of the membrane and important for cell recognition; they work like a cellular identification card.
Membrane Carbohydrates
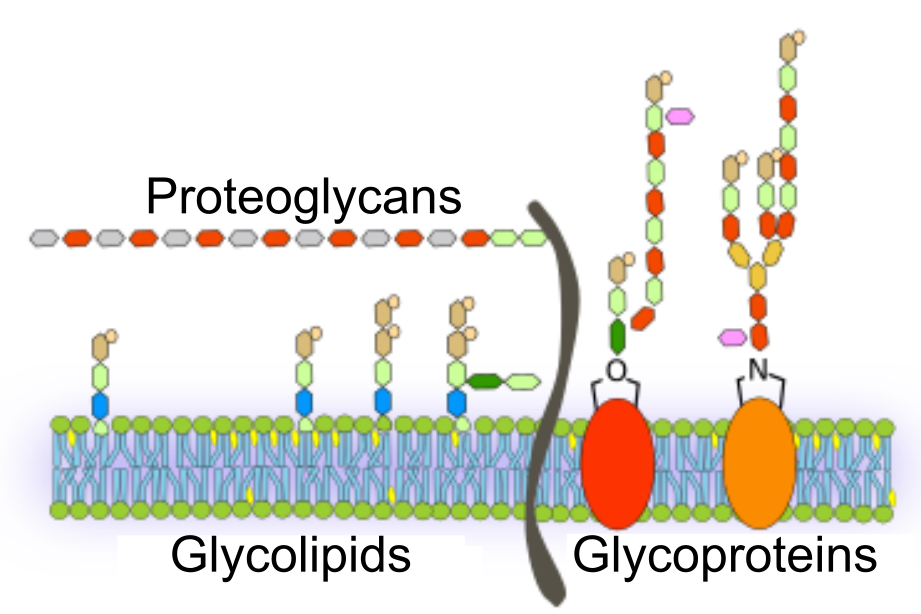
Carbohydrates are the third major plasma membrane component. They are always on the cells’ exterior surface and bound to proteins (forming glycoproteins) or lipids (forming glycolipids). These carbohydrate chains may consist of 2–60 monosaccharide units and can be either straight or branched. Along with peripheral proteins, carbohydrates form specialized sites on the cell surface that allow cells to recognize each other. These sites have unique patterns that allow for cell recognition, much like the facial features unique to each person enable individuals to recognize each other This recognition function is very important to cells, as it allows the immune system to differentiate between body cells (“self”) and foreign cells or tissues (“non-self”). Similar glycoprotein and glycolipid types are on the surfaces of viruses and may frequently change, preventing immune cells from recognizing and attacking them.
We collectively refer to these carbohydrates on the cell’s exterior surface—the carbohydrate components of glycoproteins and glycolipids—as the glycocalyx (meaning “sugar coating”). The glycocalyx is highly hydrophilic and attracts large amounts of water to the cell’s surface. This aids the cell’s interaction with its watery environment and ability to obtain substances dissolved in the water. The glycocalyx is also vital for cell identification, self/non-self determination, and embryonic development and is used in cell-to-cell attachments to form tissues.
Thus, the distribution of carbohydrates in the cell membrane is asymmetric. The extracellular face of the membrane is in contact with the extracellular fluid. The extracellular side of the membrane contains small carbohydrate groups that distinguish the cell as “self.” It also contains the end of integral proteins that interact with signals from other cells and sense the extracellular environment. The inner membrane is in contact with the cell’s contents and tends to have few carbohydrates. Instead, this intracellular side of the membrane anchors to the cytoskeleton and contains the end of integral proteins that relay signals received on the external side.
Cholesterol
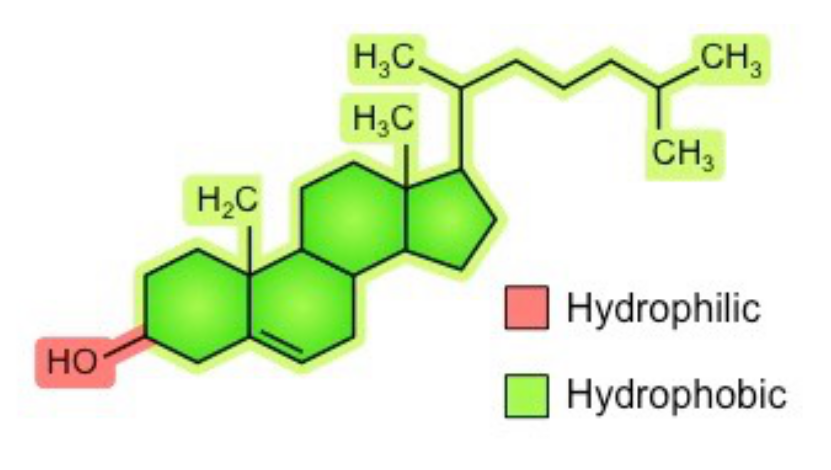
Cholesterol is a steroid and is an amphipathic molecule (like phospholipids), meaning it has both hydrophilic and hydrophobic regions. Its hydroxyl (-OH) group is hydrophilic and aligns towards the phosphate heads of phospholipids. The remainder of the molecule (steroid ring and hydrocarbon tail) is hydrophobic and associates with the phospholipid tails.
The cholesterol molecules are randomly distributed across the phospholipid bilayer, helping the bilayer stay fluid in different environmental conditions. The cholesterol holds the phospholipids together so that they don’t separate too far, letting unwanted substances in, or compact too tightly, restricting movement across the membrane. Without cholesterol, the phospholipids in your cells will start to get closer together when exposed to cold, making it more difficult for small molecules, like gases, to squeeze in between the phospholipids like they usually do. Without cholesterol, the phospholipids start to separate from each other, leaving large gaps.
Example: How Viruses Infect Specific Organs
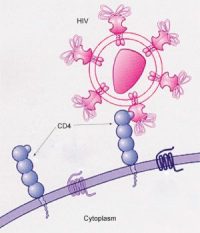
Glycoprotein and glycolipid patterns on the cells’ surfaces give many viruses an opportunity for infection. HIV and hepatitis viruses infect only specific organs or cells in the human body. HIV can penetrate the plasma membranes of a subtype of lymphocytes called T-helper cells and some monocytes and central nervous system cells. The hepatitis virus attacks liver cells.
These viruses can invade these cells because they have binding sites on their surfaces that are specific to and compatible with certain viruses. Other recognition sites on the virus’s surface interact with the human immune system, prompting the body to produce antibodies. Antibodies are made in response to the antigens or proteins associated with invasive pathogens or in response to foreign cells, such as might occur with an organ transplant. The same sites serve as places for antibodies to attach and either destroy or inhibit the virus’ activity. Unfortunately, these recognition sites on HIV change rapidly because of mutations, making an effective vaccine against the virus very difficult as the virus evolves and adapts. A person infected with HIV will quickly develop different populations, or variants, of the virus that differences in these recognition sites distinguish. This rapid change of surface markers decreases the effectiveness of the person’s immune system in attacking the virus because the antibodies will not recognize the surface patterns’ new variations. In the case of HIV, the problem is compounded because the virus specifically infects and destroys cells involved in the immune response, further incapacitating the host.
Section Summary: Membranes as Mosaics of Structure and Function
The fluid mosaic model of the cell membrane describes it as a tapestry of several types of molecules (phospholipids, cholesterols, and proteins) that are constantly moving. Membrane components move freely and fluidly in the lateral plane of the membrane. In other words, a diagram of the membrane (like the one in Figure 7.2) is just a snapshot of a dynamic process in which phospholipids and proteins are continually sliding past one another.
The biological membrane is a collage of many different proteins embedded in the fluid matrix of the lipid bilayer. The phospholipid bilayer is the main fabric of the membrane and is responsible for the fluidity of the plasma membrane. It creates a semipermeable membrane. The hydrophobic core impedes the diffusion of hydrophilic structures such as ions and polar molecules. Yet, the same structure allows small hydrophobic molecules, which can dissolve in the membrane, to cross it easily. The table below summarizes the molecules that create the mosaic and describes their locations.
Component | Location |
Phospholipid | The main fabric of the plasma membrane |
Cholesterol | Attached between phospholipids and between the two phospholipid layers |
Integral proteins | Embedded within the phospholipid layer(s); may or may not penetrate through both layers |
Peripheral proteins | On the phospholipid bilayer’s inner or outer surface, not embedded within the phospholipids |
Carbohydrates (components of glycoproteins and glycolipids) | Attached to proteins on the outside membrane layer |
Did I Get This? 7.1
Which type of lipid is most important in biological membranes?
- oils
- phospholipids
- waxes
- fats
- triglycerides
Which of the following is/are found in cell membranes? Select the single best option.
- carbohydrates
- phospholipids
- proteins
- cholesterol
- All of the above are associated with the membrane.
Which of the following is true of transmembrane proteins?
- they help with cell identification
- they are anchor points for other cells
- they have hydrophobic regions that run through the entire phospholipid bilayer
- they are important as transport proteins to move molecules in or out of the cell
- 1, 3, and 4 are correct.
The cell membrane is composed of a lipid bilayer. Is the interior of the bilayer polar or nonpolar?
- Polar
- nonpolar
Which of these are features of the fluid mosaic model of cell membranes?
- Lipid bilayer formed by amphipathic phospholipid molecules
- A mosaic formed by proteins, cholesterol, and other membrane components
- The ability for lateral diffusion of membrane components
- All of the above
Transport Across the Cell Membrane
Learning Objectives
- Define selective permeability.
- Differentiate between active and passive transport.
Selective Permeability
The plasma membrane is the boundary of the cell; it determines what enters and exits the cell and how it interacts with its environment. The cell membrane separates the extracellular and intracellular fluids, and each of these fluids contains thousands of substances. These substances often differ between the two fluids or are at least found in very different concentrations. To maintain these differences, the cells need to be selectively permeable, regulating what moves in and out. Therefore, cell membranes only allow some molecules through. This characteristic is why cell membranes are selectively permeable. They are not impermeable (meaning they do not prevent the passage of all molecules), nor are they freely permeable (meaning they don’t let all molecules freely move across the membrane). This quality allows a cell to control what enters and exits it. This section of the chapter will further explore how molecules can pass across the membrane.
Passive and Active Passage Across (Through) the Cell Membrane
There are two categories used to describe the passage of substances through a cell membrane. They are categorized as active and passive. In active transport, molecules are moved against their gradient from low- concentration to high- concentration areas. This requires the use of the cell’s energy, usually in the form of ATP. It also requires either a molecular pump within the plasma membrane or a reorganization of the plasma membrane during exocytosis and endocytosis. Passive processes do not require ATP hydrolysis but do need some driving force. This is provided by the kinetic energy of molecules when there is a concentration gradient. Molecules will move with that gradient from high to low concentrations by the random movement of molecules in passive transport mechanisms.
Learn By Doing 7.2
Define selective permeability in your own words.
Describe two differences between active and passive transport.
Passive Transport
Learning Objective
- Define diffusion.
- Describe the factors that influence the rate of diffusion.
- Provide examples of substances that move by simple diffusion and facilitated diffusion.
- Describe the process of osmosis and explain the effects of hypertonic, isotonic, and hypotonic conditions on cells and water shifts in the human body.
- Define filtration and provide examples of molecules that move across membranes via filtration.
Diffusion
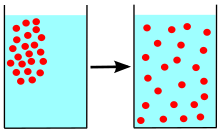
Diffusion relies on kinetic energy and a concentration gradient. Kinetic energy is affected by temperature, size of molecules, magnitude (steepness) of the gradient, and the medium (for example, the type of solution) the molecules are in. Anything that increases the molecules’ kinetic energy will increase the diffusion rate.
Diffusion is the movement of molecules from an area of high concentration to an area of lower concentration. When referring to the diffusion of molecules across a cell membrane, diffusion can be divided into simple and facilitated diffusion. In simple diffusion, the molecule passes directly through the phospholipid bilayer without the aid of a protein, whereas in facilitated diffusion, a channel or carrier protein is used. Simple diffusion occurs with either very small or lipid-soluble molecules that can pass directly through the hydrophobic region of the phospholipid bilayer of the cell membrane. Some examples of substances that use this process are oxygen (O2), carbon dioxide (CO2), and lipids. Facilitated diffusion occurs with molecules such as glucose, which may have a lower concentration inside the cell than in the extracellular space, but that requires the aid of a membrane protein for entry.
Diffusion will occur as long as there is a concentration gradient and path for movement. Once the concentration gradient no longer exists, equilibrium has been reached and net diffusion ceases. This doesn’t mean that molecules won’t move, but it does indicate that the movements are balanced in each direction, and no more changes in concentration will occur.
Diffusion most commonly occurs in gases and liquids. For example, waking up to the smell of coffee or bacon in the morning can happen because the chemicals (odorants) diffuse from the higher concentration in your kitchen to you! In the human body, the diffusion of oxygen gas (O2) and carbon dioxide gas (CO2) is critical for gas exchange. O2 levels are higher in your arterial blood than in your tissue cells, so O2 will diffuse out of the blood into your cells. CO2 has the opposite concentration gradient. CO2 levels are highest in your tissue cells (your mitochondria produce CO2 as a waste product from cellular respiration), and CO2 diffuses out of the cell into the blood. These molecules are small enough to pass through the phospholipid bilayer and are examples of simple diffusion.
Simple Diffusion Across the Plasma Membrane
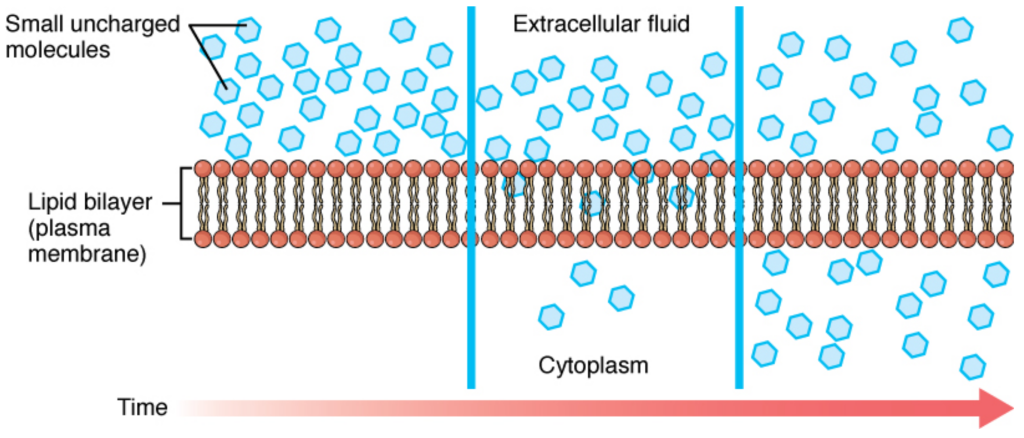
Molecules can be divided into four categories in terms of their ability to cross the plasma membrane. The first category is nonpolar molecules. These hydrophobic molecules can easily cross the membrane because they interact favorably with nonpolar lipids. Note that these molecules can accumulate in the membrane because they interact well with the lipids. The second category is small polar molecules. Although they don’t interact with the lipids, their small size allows them to pass through small temporary holes in the membrane. The third category is large polar molecules. These have difficulty crossing the membrane because of their size and poor interaction with the lipids. The last category is ionic compounds. Their charge interacts very unfavorably with the lipids, making it very difficult for them to cross the membrane.
The size, polarity, and charge of a substance will determine whether or not the substance can cross the cell membrane by diffusion. For example, even large, nonpolar substances (such as cholesterol) will freely enter the nonpolar environment of the lipid bilayer, and some will pass through to the other side. In contrast, others will stay within the cell membrane. Small amphipathic molecules such as ethanol, on the other hand, diffuse through the membrane. The lipid bilayer is much less permeable to ions because the charges are strongly excluded from the hydrophobic regions. As a general rule, charged molecules are much less permeable to the lipid bilayer.
Facilitated Diffusion
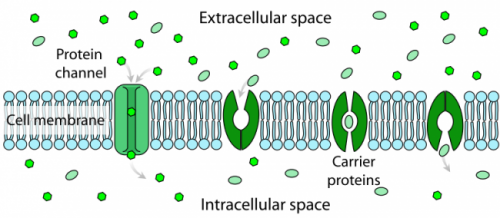
Cells must be able to move large polar and charged molecules across the lipid bilayer of the membrane to carry out life processes. To allow these molecules, which are not soluble in the lipid bilayer, to pass across the hydrophobic barrier, it is necessary to provide ports, channels, or holes through the membrane. The molecules will still move spontaneously with their concentration gradient from high to low concentration. Some of these channels can remain open at all times, allowing the molecules to move freely according to the concentration gradient. Others can be gated channels that open and close in response to the needs of the cell. In most cases, these channels are very discriminatory and will only allow specific molecules to pass. The process of moving impermeable molecules across a membrane (with their concentration gradients) using channels or pores is called facilitated diffusion. Because the molecules are moving with a concentration gradient, the process is driven by simple diffusion and does not require additional energy input from the cell.
Learn By Doing 7.3
Cells can maintain different oxygen concentrations in different organelles by using phospholipid bilayers. True or false?
- True
- False
In which situation would passive transport not use a transport protein to enter a cell?
- water flowing into a hypertonic environment
- glucose being absorbed from the blood
- an ion flowing into a nerve cell to create an electrical potential
- oxygen moving into a cell after oxygen deprivation
Which of the molecules in the image to the right would cross the membrane most easily?
Hint: To see if a molecule will pass through the membrane, you must figure out its size and polarity. To see if it is polar, remember to look for ” water-like ” parts (this means they have an OH group somewhere).
- A
- B
- C
In facilitated diffusion, molecules move through pores from areas of ____________ concentration to areas of ____________ concentration.
- high, high
- high, low
- low, high
Osmosis
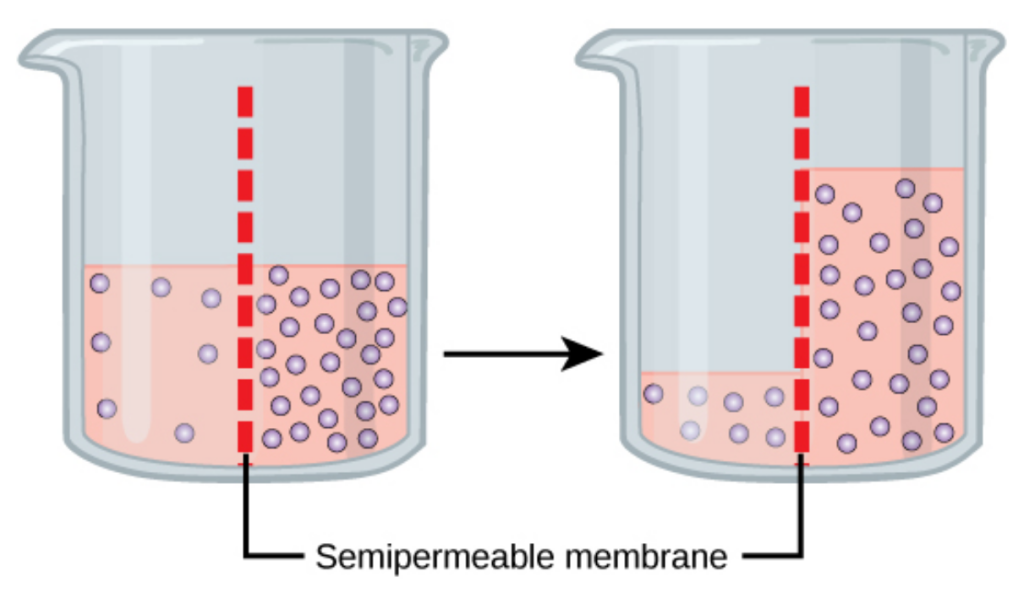
Cells continually encounter changes in their external environment. Most cells have a similar blend of solutes within them, but interstitial or extracellular fluid can vary. What will happen if a strong concentration gradient exists between a cell’s interior and the fluid outside? As you have learned, molecules tend to move down their concentration gradients until equilibrium is reached. You might think that solutes will flow into or out of the cell until the solute concentrations are equal across the membrane. However, not all molecules can pass through the cell membrane. The plasma membrane (lipid bilayer) is significantly less permeable to most solutes than to water. Therefore, the WATER tends to flow in a way that establishes an equal concentration of solutes on either side of the membrane. The water flows with its own concentration gradient, with a net movement toward the region that has a higher concentration of solutes. This movement of water across a semipermeable membrane in response to an imbalance of solute is called osmosis.
The relationship between the solute concentration and the amount of water is an inverse relationship. The more concentrated a solution is, the less water it contains. The fewer solutes, the more water – i.e., it is more dilute. Water follows gradients and moves from an area of more water to less water, but, in reality, water is moved to the area with the greater number of solutes. This creates a pressure termed osmotic pressure. Cells cannot actively move water; they must follow osmotic gradients. Solutions that have a greater solute concentration will pull water via osmotic pressure. This depends on the total number of solutes, not the type. Note that some water can pass through the cell membrane, but most water passes through protein membrane channels termed aquaporins.
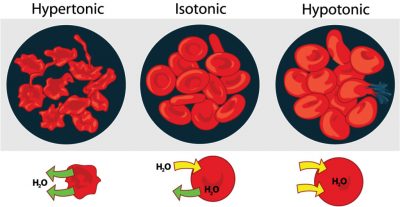
Cells may find themselves in three different sorts of solutions. The terms isotonic, hypertonic, and hypotonic refer to the concentration of solutes outside the cell relative to the solute concentration inside the cell. In an isotonic solution, solutes and water are equally concentrated within and outside the cell. 0.9% NaCl (physiologic saline) and 5% dextrose are two standard isotonic solutions. The cell is bathed in a solution with a solute concentration similar to its cytoplasm. Many medical preparations (saline solutions for nasal sprays, eye drops, and intravenous drugs) are designed to be isotonic to our cells. A hypotonic solution has a low solute concentration and a high concentration of water compared to the cell’s cytoplasm. Distilled (pure) water is the ultimate hypotonic solution as, by definition, it contains no solutes. If a cell is placed in a hypotonic solution, it will tend to gain water. The solutes will “stay put” within the cell, but water molecules will diffuse such that their net flow is toward the area with a higher concentration of solutes. A hypertonic solution has a high solute concentration (lower water concentration) compared to the cell cytoplasm. Very salty or sugary solutions (brines or syrups) are hypertonic to living cells. If a cell is placed in such a solution, water tends to flow spontaneously out of the cell.
Filtration
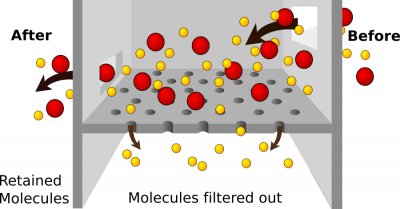
Filtration is another passive process of moving material through a cell membrane. While diffusion and osmosis rely on concentration gradients, filtration uses a pressure gradient. Molecules will move from an area of higher pressure to an area of lower pressure. Filtration is non-specific. This means that it doesn’t sort the molecules; they pass due to pressure gradients and their size. If molecules are small enough to pass through the membrane, they will. The force that pushes the molecules is termed hydrostatic pressure.
One example of filtration is making coffee. Think of the coffee filter as the cell membrane and the coffee grounds, flavor, and caffeine as the molecules. The pressure is exerted by gravity acting on water from the machine. It forces materials through the coffee filter into the coffee pot. Small molecules like caffeine, water, and flavor pass through the filter, but the coffee grounds do not. They are too big. If you poke holes in the filter, the coffee grounds would end up in your coffee! The coffee filter represents the filtration membrane which is typically a layer of cells.
Filtration is one of the main methods used for capillary exchange. Blood pressure provides the driving force (hydrostatic pressure) to force materials out of capillaries and into the tissue fluid surrounding cells. It is also the process used to form the filtrate that begins the urine formation in the kidney. Hydrostatic pressure is countered by osmotic pressure. Remember that osmotic pressure is created due to increased solute concentration and will pull water toward the area of higher solute concentration. These two pressures must be in balance for the homeostasis of fluid volumes. In our body, large molecules such as plasma proteins and red blood cells should not pass out of the blood through the cell membranes lining the capillaries. If these substances pass through and end up in tissue fluid or the kidney and later the urine, it is abnormal and a sign of disease.
Learn By Doing 7.4
Water moves via osmosis _________.
- throughout the cytoplasm
- from an area with a high concentration of other solutes to a lower one
- from an area with a high concentration of water to one of a lower concentration
- from an area with a low concentration of water to a higher concentration
The principal force driving movement in diffusion is __________.
- temperature
- particle size
- the concentration gradient of the molecule being considered
- membrane surface area
Both of the regular intravenous solutions administered in medicine, normal saline and lactated Ringer’s solution, are isotonic. Why is this important?
Imagine a solution in beaker A is 20% glucose, and the solution in beaker B is 40% glucose; which beaker is hypertonic?
Hint: Remember the prefix “hyper” means too much.
If you connected the beakers described above with a tube that was permeable to water but impermeable to glucose, which way would water move?
Hint: Only water can pass through the membrane. Which solution has a higher osmolarity (think the concentration of solute) to pull water?
- From beaker A to beaker B
- From beaker B to beaker A
- Water would not move
Human cells have an internal solute concentration of approximately 1%. This solute concentration includes salts, sugars, proteins, and all the other molecules dissolved in the cytoplasm. What is the internal water concentration of a cell with an internal solute concentration of 1%?
Hint: The concentration of solute + water = 100%.
- 1%
- 99%
- 100%
- It is not possible to determine from the information given.
What external solute concentration is isotonic to the cell above?
Hint: Isotonic means the cell and environment have the same solute concentration.
- 1%
- 99%
- 100%
In an isotonic situation, which way will water flow?
Hint: Water moves from high concentration to low concentration.
- Mostly out of the cell.
- Mostly into the cell.
- Equally into and out of the cell.
Nemo is a clownfish, and clownfish live in the ocean. The salinity of Nemo’s ocean environment averages around 3.5%. What would happen to Nemo if he became lost and ended up in a freshwater lake?
Hint: Think about Nemo’s natural habitat and how his body has adapted.
- Nemo would die because his cells would lose water, and he would dehydrate quickly.
- Nemo would swell and could pop if he didn’t get out of there.
- Nemo would be fine; there wouldn’t be any water movement.
Which way does water move when an individual is dehydrated?
A patient has had a stomach virus for two days and has complained of vomiting and diarrhea. You are very concerned about dehydration and realize that the patient is severely dehydrated. Which fluid (water) shifts occur in the patient’s tissue cells?
- Water enters the patient’s cells from the blood.
- Water is pulled out of the patient’s cells and into the blood.
- Dehydration does not affect water movement in or out of cells.
If trying to reduce edema, what type of IV would be given?
Another patient presents with edema due to acute hepatitis infection. To clear the excess fluid, what type of IV would you give? Note that edema is increased water in the interstitial (extracellular fluid) that bathes cells.
- Isotonic
- Hypertonic
- Hypotonic
Active Transport
Learning Objectives:
- Describe the process of active transport, its energy requirements, and list examples of substances that use it.
- Describe endocytosis and exocytosis as a means of moving materials across the membrane.
You have just finished investigating the passive methods of transport. Now let’s look at active transport. In active methods, the cell must expend energy (ATP) to do the work of moving molecules. Active transport often occurs when the molecule is being moved against its concentration gradient or when moving very large molecules into or out of the cell. There are two main types of active processes.
- Primary Active Transport or Solute Pumps
- Bulk Transport via Endocytosis and Exocytosis
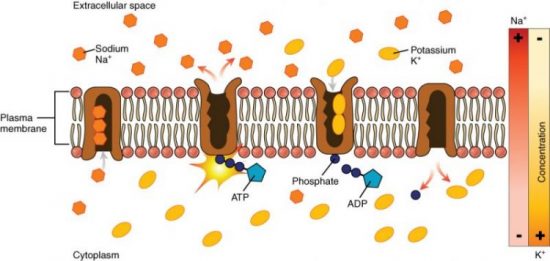
One form of active transport involves moving ions from an area of low concentration to an area of higher concentration. If you need to roll a rock, it’s much easier to roll it downhill rather than uphill, right? Active transport is moving the rock uphill, and this requires energy. The best example is the sodium (Na+)/potassium (K+) exchange pump. The interior of a cell contains a low Na+ concentration compared to the extracellular fluid. The interior of the cell (intracellular fluid or ICF) contains more K+ than the extracellular fluid (ECF). This creates an imbalance in these ions across the cell membrane. Na+ has a concentration gradient to enter the cell since there is more Na+ in the extracellular fluid. K+ has a concentration gradient for it to leave the cell. The cell membrane is not impermeable to these ions, and some of them escape following their concentration or diffusion gradients. You will later study why it is essential to maintain these ion concentrations. The Na+/K+ pump is an active transport pump that moves Na+ “uphill” back out of the cell against its concentration gradient and simultaneously moves K+ back into the cell against its concentration gradient. This pump requires ATP hydrolysis, a membrane protein transporter, and enzymes to function.
Endocytosis and Exocytosis
Facilitated diffusion and active transport are not the only ways that materials can enter or leave cells. Through the processes of endocytosis and exocytosis, large amounts of material can be taken up or ejected in bulk without passing through the cell’s plasma membrane. This is referred to as bulk transport.
In endocytosis, material is engulfed within an infolding of the plasma membrane and then brought into the cell within a cytoplasmic vesicle. To begin endocytosis, a particle encounters the cell surface and produces a dimple or pit in the membrane. The pit deepens, invaginates further, and finally pinches off to form a vesicle in the cell’s cytoplasm. During the process, the inside surface of the newly formed vesicle is the same as the exterior surface of the cell. Thus, the chemistry of the cytoplasm and the orientation of the plasma membrane are maintained. Once internalized, this new vesicle containing extracellular materials may fuse with a lysosome so that its solid contents are digested. The resulting molecules may be released to the cytoplasm for use within the cell.
There are two general forms of endocytosis: phagocytosis and pinocytosis. Phagocytosis is the uptake of large solid particles such as bacteria or cellular debris. Pinocytosis is the uptake of fluid and any small molecules dissolved within it. Cells can also recognize specific particles and engulf them in a more targeted way, a process called receptor-mediated endocytosis. In this case, the particle first binds to a membrane protein receptor on the cell’s surface. The binding of the target particle induces the cell to engulf it.
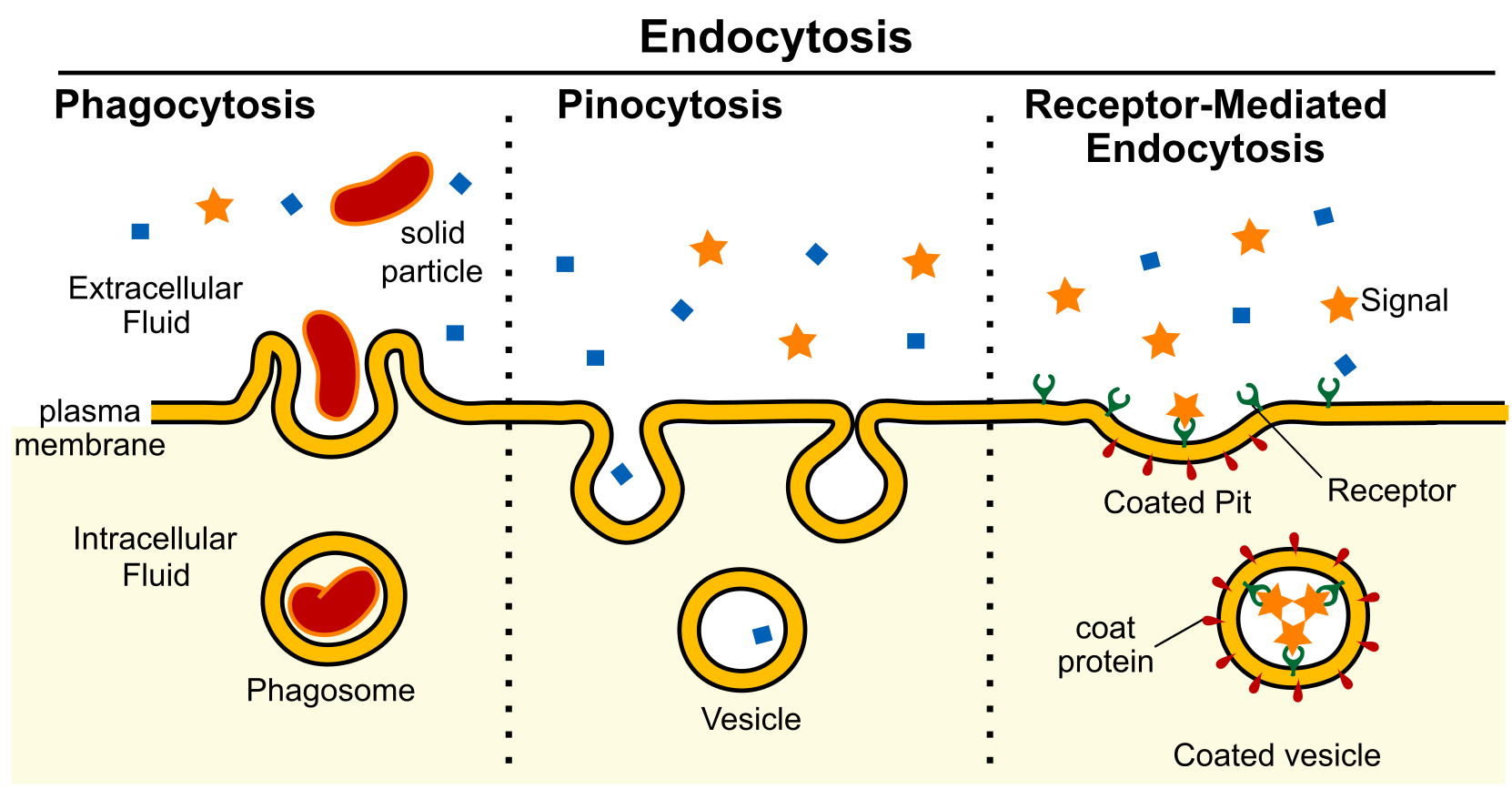
Exocytosis is the reverse of endocytosis. Its purpose is to expel material from the cell into the extracellular fluid, replenish the plasma membrane, and allow the cell to respond to signals from outside the cell. Constitutive secretion is a continuous form of exocytosis, and all cells perform it. (Constitutive in this context means essential.) This most common form of exocytosis is the mechanism cells use to maintain the plasma membrane. Newly formed proteins are embedded in vesicles created with newly synthesized phospholipids. The vesicles are delivered to the membrane and fuse with it. Vesicles may contain proteins or carbohydrates that make up the extracellular matrix. Constructive exocytosis also allows cells to respond to their environment by altering which membrane proteins are present on the cell surface. In addition, the cell rids itself of waste with this mechanism. Waste material is enveloped in a membrane and fuses with the plasma membrane’s interior. This fusion opens the membranous envelope on the cell’s exterior, and the waste material expels into the extracellular space.
Regulated exocytosis allows the build-up of vesicles near the plasma membrane. Vesicles may contain secretory proteins such as digestive enzymes, hormones, or neurotransmitters. Fusion of vesicles is triggered when a specific signal is received from outside the cell.
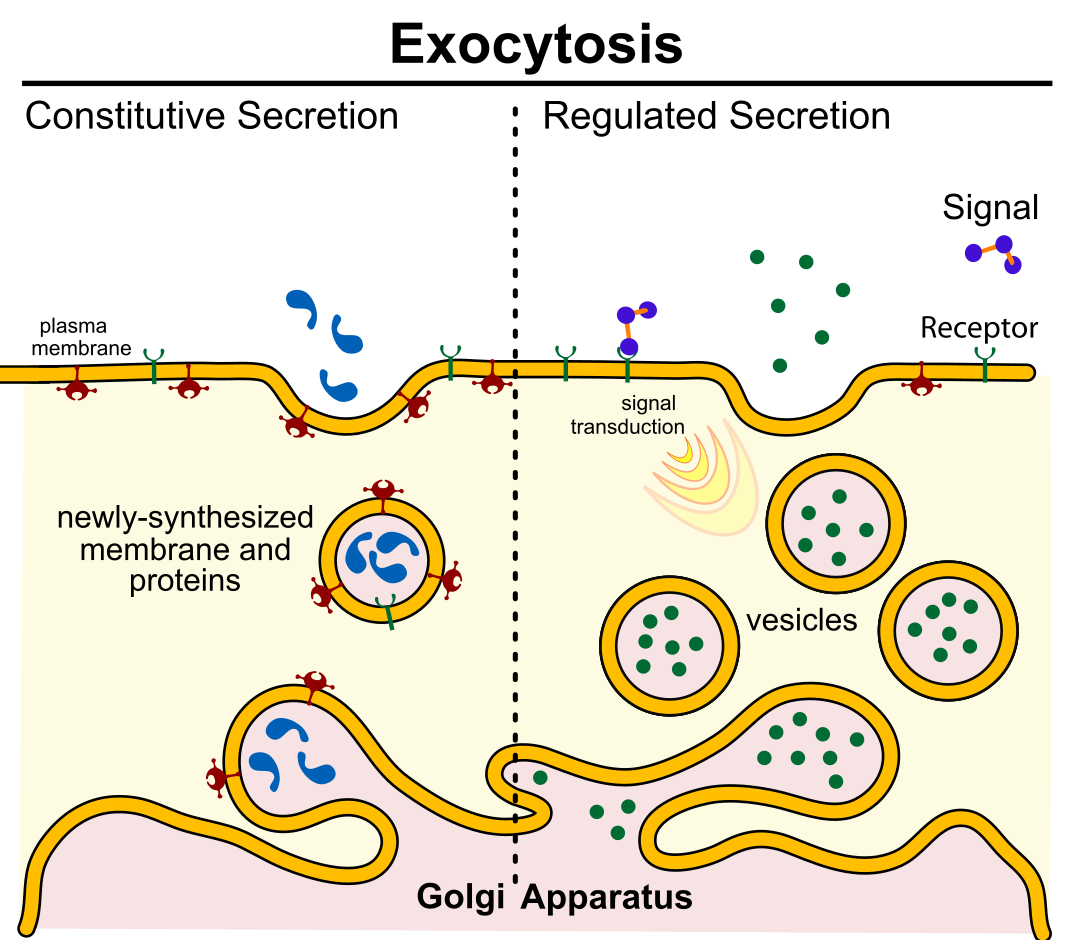
The balance of exocytosis and endocytosis preserves the size of the plasma membrane and keeps the cell’s size constant. How are endocytosis and exocytosis important to everyday life? Immune cells protect animals by recognizing and destroying foreign objects such as bacteria. Disease-causing bacteria are recognized by proteins called receptors on the immune cell’s surface. The phagocytic immune cell will then engulf the bacterial cells (phagocytosis). The vesicle that contains these bacterial cells is called a phagosome (“phago” means “eating,” and “-some” refers to “body”). The phagosome next fuses with lysosomes. Finally, the digested bacterial products are excreted through the process of exocytosis. Exocytosis is employed for the secretion of hormones and the release of neurotransmitters. Both of these processes are critical for homeostasis.
Section Summary
The passive transport forms, simple diffusion, facilitated diffusion, and osmosis, move small molecular weight materials across membranes. Substances diffuse from high to lower concentration areas, and this process continues until the substance evenly distributes itself in a system. In solutions containing more than one substance, each molecule type diffuses according to its concentration gradient, independent of other diffusing substances. Many factors can affect the diffusion rate, such as concentration gradient, diffusing, particle sizes, and the system’s temperature.
Active transport methods require directly using ATP to fuel the transport. In a process scientists call phagocytosis, other cells can engulf large particles, such as macromolecules, cell parts, or whole cells. In phagocytosis, a portion of the membrane invaginates and flows around the particle, eventually pinching off and leaving the particle entirely enclosed by a plasma membrane’s envelope. The cell breaks down vesicle contents, using the particles either as food or exporting them as waste. Pinocytosis is a similar process on a smaller scale. The plasma membrane invaginates and pinches off, producing a small envelope of fluid from outside the cell. Pinocytosis imports substances that the cell needs from the extracellular fluid. The cell expels waste in a similar but reverse manner. It pushes a membranous vacuole to the plasma membrane, allowing the vesicle to fuse with the membrane and incorporate itself into the membrane structure, releasing its contents to the exterior.
In living systems, the plasma membrane mediates substances diffusing in and out of cells. Some materials diffuse readily through the membrane, but others are hindered and only can pass through due to specialized proteins such as channels and transporters. The chemistry of living things occurs in aqueous solutions, and balancing the concentrations of those solutions is an ongoing problem. In living systems, diffusing some substances would be slow or difficult without membrane proteins that facilitate transport.
The table below summarizes the different methods of transport across the plasma membrane.
Transport Method | Active/Passive | Material Transported |
Diffusion | Passive | Small-molecular weight material |
Osmosis | Passive | Water |
Facilitated diffusion | Passive | Sodium, potassium, calcium, glucose |
Active transport | Active | Sodium, potassium, calcium, amino acids, lactose |
Phagocytosis | Active | Large macromolecules, whole cells, or cellular structures |
Pinocytosis | Active | Small molecules (liquids/water) |
Exocytosis | Active | Neurotransmitters, hormones |
Learn by Doing 7.5
Active transport must function continuously because __________.
- plasma membranes wear out
- not all membranes are amphiphilic
- facilitated transport opposes active transport
- diffusion is constantly moving solutes in opposite directions
How does the sodium-potassium pump make the interior of the cell negatively charged?
Hint: Remember that anions are negatively charged, and cations are positively charged.
- by expelling anions
- by pulling in anions
- by expelling more cations than are taken in
- by taking in and expelling an equal number of cations
What happens to the membrane of a vesicle after exocytosis?
- It leaves the cell.
- It is disassembled by the cell.
- It fuses with and becomes part of the plasma membrane.
- It is used again in another exocytosis event.
Which transport mechanism can bring whole cells into a cell?
- pinocytosis
- phagocytosis
- facilitated transport
- primary active transport
Where does the cell get energy for active transport processes?
Why is it essential that there are different types of proteins in plasma membranes to transport materials into and out of a cell?
*
“Learn By Doing” and “Did I Get This?” Feedback
Learn By Doing 7.1
Describe the functions of the plasma membrane.
The cell membrane has many functions, each made possible by specific molecules forming it.
- It defines the cell, isolating the chemistry inside it from outside it.
- It determines the nature of its interaction with its environment by allowing specific molecules to move in and keeping others out.
- It flexibly outlines its borders, allowing shape changes if cells move and as they pass through narrow capillaries
- It allows cells to recognize one another and plays a part in development and immune responses.
- It allows a cell to transmit and receive signals from other cells.
Membranes are described in all of the following ways except
- semipermeable barriers
Incorrect. It is true that membranes are semipermeable. - are fluid structures dependent on cholesterol and saturated fat composition
Incorrect. This is a description of membranes; these characteristics determine the membrane’s fluidity. - will allow the transport of ions through the lipid layer
Correct. Polar and charged particles cannot pass directly through the lipid layer of the membrane. - uniform
Incorrect. Membranes are fairly uniform in structure – they always have a double layer of phospholipids. - lipid bilayer
Incorrect. This is a characteristic of membranes.
Did I Get This? 7.1
Which type of lipid is most important in biological membranes?
- oils
- phospholipids
- waxes
- fats
- triglycerides
Which of the following is/are found in cell membranes? Select the single best option.
- carbohydrates
- phospholipids
- proteins
- cholesterol
- All of the above are associated with the membrane.
Which of the following is true of transmembrane proteins?
- they help with cell identification
- they are anchor points for other cells
- they have hydrophobic regions that run through the entire phospholipid bilayer
- they are important as transport proteins to move molecules in or out of the cell
- 1, 3, and 4 are correct.
The cell membrane is composed of a lipid bilayer. Is the interior of the bilayer polar or nonpolar?
- Polar
Incorrect. The phosphate heads, which are polar, are on the outsides of the bilayer, not the interior. - nonpolarCorrect. The fatty acid side chains, which are nonpolar, are in the interior of the bilayer.
Which of these are features of the fluid mosaic model of cell membranes?
- Lipid bilayer formed by amphipathic phospholipid molecules
- A mosaic formed by proteins, cholesterol, and other membrane components
- The ability for lateral diffusion of membrane components
- All of the above
According to the fluid mosaic model, the plasma membrane is a lipid bilayer and mosaic of phospholipids, cholesterol, and proteins—that move freely and fluidly in the plane of the membrane.
Learn By Doing 7.2
Define selective permeability in your own words.
Selectively permeable/semipermeable means that the cell membrane can only be freely crossed by certain molecules, namely those that are small and nonpolar. The hydrophobic inner part of the membrane prevents ions and polar molecules from entering the cell. Polarity and size are the more important molecular features that influence the ability to cross membranes.
Describe two differences between active and passive transport.
Active transport requires the cell to use its own energy, moves molecules from areas of high to low concentration (against their gradient), and requires either a molecular pump or reorganization of the membrane. Passive transport is powered by kinetic energy and a concentration gradient and moves molecules with the concentration gradient.
Learn By Doing 7.3
Cells can maintain different oxygen concentrations in different organelles by using phospholipid bilayers. True or false?
- True
- False, This is not possible since oxygen is small and nonpolar, so it is impossible to prevent its passage across the membrane.
In which situation would passive transport not use a transport protein for entry into a cell?
- water flowing into a hypertonic environment
- glucose being absorbed from the blood
- an ion flowing into a nerve cell to create an electrical potential
- oxygen moving into a cell after oxygen deprivation
Which of the molecules shown to the right would cross the membrane most easily?
Hint: To see if a molecule will pass through the membrane, you have to figure out its size and polarity. To see if it is polar, remember to look for ” water-like ” parts (this means they have an OH somewhere).
- A, incorrect. Glucose is a large polar molecule that requires a transport protein.
- B, incorrect. The chloride ion carries a charge and is repelled by the hydrophobic core of the cell membrane.
- C, correct. CH4 is a small, nonpolar molecule. Glucose has many OH (hydroxyl) groups, making it polar and Cl– carries a charge. (Even if we didn’t know the molecule’s polarity, we can tell this is the correct choice by process of elimination.)
In facilitated diffusion, molecules move through pores from areas of ____________ concentration to areas of ____________ concentration.
- high, high
incorrect. Diffusion of any kind requires the potential energy provided by a difference in concentration. - high, low
Correct. Diffusion is defined as molecules moving from an area of high concentration to an area of low concentration.
- low, high
Incorrect. The use of ATP and a membrane pump would be required to move molecules from areas where there are fewer to areas where there are more.
Learn by Doing 7.4
Water moves via osmosis _________.
- throughout the cytoplasm
- from an area with a high concentration of other solutes to a lower one
- from an area with a high concentration of water to one of a lower concentration
- from an area with a low concentration of water to a higher concentration
The principal force driving movement in diffusion is the __________.
- temperature
- particle size
- concentration gradient of the molecule being considered
- membrane surface area
In which situation would passive transport not use a transport protein for entry into a cell?
- water flowing into a hypertonic environment
- glucose being absorbed from the blood
- an ion flowing into a nerve cell to create an electrical potential
- oxygen moving into a cell after oxygen deprivation
Both of the regular intravenous solutions administered in medicine, normal saline and lactated Ringer’s solution, are isotonic. Why is this important?
Our Answer: Injection of isotonic solutions ensures that there will be no perturbation of the osmotic balance and no water taken from tissues or added to them from the blood.
The solution in beaker A is 20% glucose, and the solution in beaker B is 40% glucose; which beaker is hypertonic?
Hint: Remember the prefix “hyper” means too much.
- Beaker A, Incorrect. The more solutes in a solution, the higher its percentage.
- Beaker B, Correct. A 40% glucose solution contains more glucose than a 20% glucose solution.
If you connected beakers A and B from above with a tube that was permeable to water but impermeable to glucose, which way would water move?
Hint: Only water can pass through the membrane. Which solution has a higher osmolarity (think the concentration of solute) to pull water?
- From beaker A to beaker B
Correct. The hypertonic solution in B would pull water toward it. Water always flows from hypotonic to hypertonic areas. - From beaker B to beaker A
Incorrect. Beaker A has fewer solutes than beaker B. - Water would not move.
Incorrect. The fluids are not isotonic; water will move to the hypertonic fluid. In fact, water molecules will move even between 2 isotonic solutions. However, there will be no net movement of water.
Human cells have an internal solute concentration of approximately 1%. This solute concentration includes salts, sugars, proteins, and all the other molecules dissolved in the cytoplasm. What is the internal water concentration of a cell with an internal solute concentration of 1%?
Hint: The concentration of solute + water = 100%.
- 1%
Incorrect. This is the internal solute concentration. - 99%
Correct. If there is 1% solute in a solution, the other 99% must be water! - 100%
Incorrect. This is the total concentration of solute + water. - It is not possible to determine from the information given.
Incorrect. The solute concentration + water concentration = 100%
What external solute concentration is isotonic to the cell above?
Hint: Isotonic means the cell and environment have the same solute concentration.
- 1%
Correct. A solution that is isotonic to this cell will have the SAME concentration as the cell! - 99%
Incorrect. This is the concentration of water. What is the concentration of solute? - 100%
Incorrect. This is the total concentration of solute + water.
In an isotonic situation, which way will water flow?
Hint: Water moves from high concentration to low concentration.
- Mostly out of the cell.
Incorrect. Water tends to move with its own concentration gradient. But in an isotonic solution, there is no gradient: solute concentrations outside and inside the cell are equal. - Mostly into the cell.
Incorrect. Water tends to move with its own concentration gradient. But in an isotonic solution, there is no gradient: solute concentrations outside and inside the cell are equal. - Equally into and out of the cell.
Correct. Solute concentrations are equal inside and outside the cell. There will be no net flow of water; water molecules will move at equal rates in and out of the cell.
Nemo is a clownfish, and clownfish live in the ocean. The salinity of Nemo’s ocean environment averages around 3.5%. What would happen to Nemo if he became lost and ended up in a freshwater lake?
Hint: Think about Nemo’s natural habitat and how his body has adapted to it.
- Nemo would die because his cells would lose water, and he would dehydrate quickly.
Incorrect. Remember, Nemo is moving from a salty environment into a low-salt environment. - Nemo would swell and could pop if he didn’t get out of there.
Correct. Poor Nemo! His body cells are used to the higher salinity and are hypertonic to his freshwater environment. - Nemo would be fine; there wouldn’t be any water movement.
Incorrect. Water must follow osmotic gradients, and there is a gradient between Nemo’scells and the freshwater lake.
Which way does water move when an individual is dehydrated.? A patient has had a GI bug for two days and has complained of vomiting and diarrhea. You are very concerned about dehydration and realize that the patient is severely dehydrated. Which fluid (water) shifts are occurring in the patient’s tissue cells?
- Water enters the patient’s cells from the blood.
Incorrect. The patient has lost water due to the GI virus; therefore, the blood is less dilute and contains less water.
- Water is being pulled out of the patient’s cells into the blood.
Correct. The water loss from the patient has created hypertonic blood, which pulls water from the cells (and tissue [interstitial] fluid).
- Dehydration does not affect water movement in or out of cells.
Incorrect. Water will move – think about the patient’s blood. Is it too dilute or too concentrated?
If trying to reduce edema, what type of IV would be given? Another patient presents with edema due to acute hepatitis infection. To clear the excess fluid, what kind of IV would you give? Note edema is an increase in water in the interstitial (extracellular fluid) that bathes cells.
- Isotonic
Incorrect. An isotonic IV solution would maintain the current conditions, not remove the excess water. - Hypertonic
Correct. The hypertonic IV would pull water into the blood from the interstitial space to help clear the edema. This excess water will travel via the blood to the kidneys, where it can be excreted as urine. - Hypotonic
Incorrect. This could kill your patient! Hypotonic IV solutions will increase water loss from the blood into the interstitial space and cells.
Learn by Doing 7.5
Active transport must function continuously because __________.
- plasma membranes wear out
- not all membranes are amphiphilic
- facilitated transport opposes active transport
- diffusion is constantly moving solutes in opposite directions
How does the sodium-potassium pump make the interior of the cell negatively charged?
- by expelling anions
- by pulling in anions
- by expelling more cations than are taken in
- by taking in and expelling an equal number of cations
What happens to the membrane of a vesicle after exocytosis?
- It leaves the cell.
- It is disassembled by the cell.
- It fuses with and becomes part of the plasma membrane.
- It is used again in another exocytosis event.
Which transport mechanism can bring whole cells into a cell?
- pinocytosis
- phagocytosis
- facilitated transport
- primary active transport
Where does the cell get energy for active transport processes?
The cell harvests energy from ATP produced by its own metabolism to power active transport processes, such as the activity of pumps.
Why is it essential that there are different types of proteins in plasma membranes to transport materials into and out of a cell?
The proteins allow a cell to select what compound will be transported, meeting the needs of the cell and not bringing in anything else. This adds additional selectivity to the plasma membranes.
Media Attributions
- Grand Central © Open Stax is licensed under a CC BY (Attribution) license
- membrane diagram © Open Stax is licensed under a CC BY (Attribution) license
- membrane-protein-functions_med
- membrane protein types © B. Cornell (BioNinja) is licensed under a CC BY-NC-SA (Attribution NonCommercial ShareAlike) license
- membrane carbohydrates © Dpt. of Functional Biology and Health Sciences. Faculty of Biology. University of Vigo. Spain
- cholesterol © B. Cornell (BioNinja)
- 220px-Diffusion.svg
- simple diffusion © Open Stax is licensed under a CC BY (Attribution) license
- Scheme_facilitated_diffusion_in_cell_membrane-en
- cross_membrane_molecules_DIGT
- osmosis beaker © Open Stax is licensed under a CC BY (Attribution) license
- Figure_41_05_01-1024×530
- Filtration_diagram
- 0308_Sodium_Potassium_Pump
- membrane reorganizartion © Mariana Ruiz Villarreal
- Exocytosis_types © Mariana Ruiz is licensed under a Public Domain license