Support Systems of the Body
10 Muscular System
Introduction to the Muscular System
When you think of muscles, you probably have a picture in your mind of some muscular people who are pleasing to the eye. Did you know that you have over 650 muscles in your body? That sounds like a lot, but a caterpillar has around 4000 muscles! Without muscles, we would never be able to move. The main function of the muscular system is movement. But did you know that muscle contractions generate 85% of our body heat? Muscles also protect our organs. Your abdominal muscles keep your “guts” and internal organs in place. Some muscles are circular, such as the one that encircles your mouth for kissing or puckering. Other circular muscles form sphincters that control when you defecate or urinate. One important muscle, known as your diaphragm, is necessary for breathing. If your diaphragm stopped working you would die in minutes because you would not breathe.
Learn By Doing 11.1
Let’s test your knowledge of some common facts and myths about the muscular system. Decide whether the following statements are fact or myth.
- If you eat a lot of protein, you will store it in your muscles, and they will get bigger.
- It takes more muscles to frown than to smile
- The muscle (based on weight) that can generate the most pressure in the human body is the tongue.
- The hardest working muscle in the body is the heart.
- Skeletal muscle is 75% water.
The muscular system is mainly composed of skeletal muscles, such as those that cover the anterior portion of your abdomen and help compress the abdominopelvic cavity and digestive organs. If these muscles are weakened, they might separate and allow the underlying organs to protrude. The small intestine is a critical component of the digestive system and if it is pinched off that section could become necrotic and die.
There are two other types of muscle tissue that are necessary for life: cardiac and smooth muscle. Cardiac muscle is found in the heart, and its rhythmic contraction is responsible for your heartbeat, while smooth muscle is found in many organs and blood vessels. Smooth muscle is a significant part of your cardiovascular system (blood vessels), respiratory system (bronchioles), digestive system (esophagus, stomach, small and large intestines), urinary system (ureters and urinary bladder), and reproductive system (uterus, vas deferens).
As stated earlier, skeletal muscle has many important functions in maintaining homeostasis. Skeletal muscle contractions are critical in producing heat for our body. In fact, when you are very cold, your skeletal muscles increase contractions to generate more heat to warm you – this is known as shivering. When you exercise and your skeletal muscles are more active, you produce more body heat, and your body temperature increases. Your body will sweat to help cool itself.
The diaphragm is a skeletal muscle that contracts to allow us to inhale; we exhale when it relaxes. Any disruption in this important muscle’s function can be fatal. The diaphragm is a critical organ of the respiratory system.
Clearly, skeletal muscle and muscle tissue are much more important than just giving us a “buff” body!
Muscular System Structures and Functions
Learning Objectives
- Identify parts of the muscular system.
- Differentiate between skeletal, cardiac, and smooth muscle in terms of structure, location, and function.
The muscular system consists of skeletal muscle connected to bones by tendons, a type of dense regular connective tissue that joins muscles to bone. The abdominal muscles are also held with broad tendon sheaths termed aponeuroses.
As mentioned, there are three types of muscle tissue. Skeletal muscle attaches to bones, is voluntary, and has a striped (striated) appearance. Cardiac muscle is found in the heart, is involuntary, and is striated. Smooth muscle, sometimes known as visceral muscle, is found in many organs and blood vessels, is not striated, and is involuntary.
Muscles allow for movement such as walking, and they also facilitate bodily processes such as respiration and digestion. The body contains three types of muscle tissue: skeletal muscle, cardiac muscle, and smooth muscle.
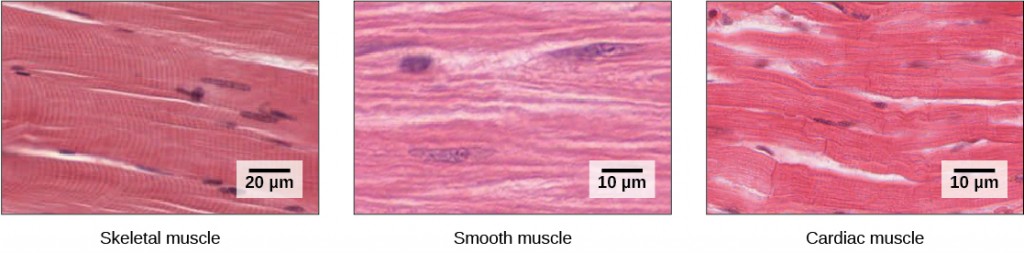
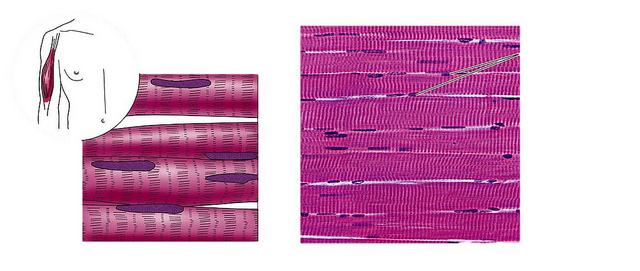
Skeletal muscle tissue forms skeletal muscles, which attach to bones and sometimes the skin and control locomotion and any other movement that can be consciously controlled. Because it can be controlled intentionally, skeletal muscle is also called voluntary muscle. When viewed under a microscope, skeletal muscle tissue has a striped or striated appearance. This appearance results from the arrangement of the proteins inside the cell that are responsible for contraction. The cells of skeletal muscle are long and tapered and have multiple nuclei on the periphery of each cell.
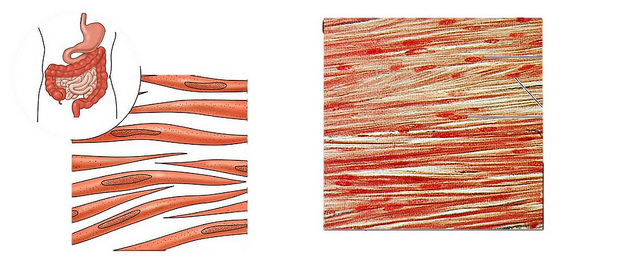
Smooth muscle tissue occurs in the walls of hollow organs such as the intestines, stomach, and urinary bladder and around passages such as in the respiratory tract and blood vessels. Smooth muscle has no striations, is not under voluntary control, and is called involuntary muscle. Smooth muscle cells have a single nucleus.
Cardiac muscle tissue is only found in the heart. The contractions of cardiac muscle tissue pump blood throughout the body and maintain blood pressure. Like skeletal muscle, cardiac muscle is striated, but unlike skeletal muscle, cardiac muscle cannot
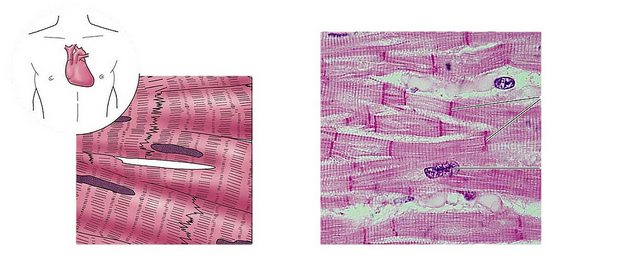
be consciously controlled and is called involuntary muscle. The cells of cardiac muscle tissue are connected to each other through intercalated disks and usually have just one nucleus per cell.
Muscle Physiology: The Big Picture
How does a muscle contract? In order to answer this question, we must first examine what tells a muscle to contract. Let’s say that I am sitting here writing and want to pick up a cup of coffee. In order to do so, I must send a command to the muscles in my arm. The command comes from a thought generated in my nervous system. The command travels from my brain to my spinal cord to a nerve that attaches to a muscle in my arm. My nervous system communicates with my skeletal muscles via acetylcholine, a chemical messenger known as a neurotransmitter. The command tells my muscle to contract, and my arm dutifully responds by moving closer to the coffee.
Muscle cells, like all cells, are made of biological macromolecules. If we were to examine a skeletal muscle under a microscope, we would see that it is composed of many tiny protein fibers or filaments made primarily of the proteins actin and myosin. When a muscle receives a command from the nervous system to contract, the protein filaments slide past each other. In fact, one of the filaments connects to the other and drags it along. Think of thousands of overlapping filaments sliding past each other as the muscle contracts. The command to contract must somehow get from the outside of the muscle to the inside. Tiny messengers called neurotransmitters bring the message from the nerve to the muscle. Other chemical messengers that tell the protein filaments to contract then pass on the message. Muscles need energy to contract. Muscles must have a power source in order to power the sliding filaments. The energy comes from ATP. One type of filament binds ATP and uses energy from ATP breakdown to pull the other filament along.
Skeletal muscle fibers or cells are not all alike. There are cells that are specialized for endurance events, known as slow-twitch fibers. There are also cells or fibers that are better suited for power and sprinting. These fatigue a lot faster and are known as fast-twitch fibers.
As you study the muscular system, you will learn about the molecular and microscopic anatomy and physiology of muscle. This is necessary in order to understand how muscle contracts. You will also study the gross anatomy of muscles, their names, locations, and functions in the human body. The muscular system is a fascinating system. Enjoy the journey as you discover it!
Learn By Doing 11.2
True or false: All muscle tissue is formed from muscle fibers and is striated.
Hint: The name of the muscle tissue gives important information regarding its appearance.
Which ion is necessary for muscle contraction?
- acetylcholine
- potassium
- calcium
- myosin
Hint: This ion is critical for force generation in muscle.
Skeletal Muscle Function
Learning Objective
Skeletal muscles (organs) are almost never completely relaxed. Even if a muscle is not producing movement, some of the muscle fibers (cells) in the organ contract to produce muscle tone. The tension produced by muscle tone prevents the muscle from becoming weak, allowing the muscle to stabilize joints and maintain posture. Muscle tone is accomplished by the contraction of a small fraction of muscle cells so muscles won’t fatigue completely; some cells can recover while others are active. This can help with stronger movements, as the motor units being used to maintain tone can produce a quicker, stronger contraction than the motor units that are resting.
The absence of the low-level contractions that lead to muscle tone is referred to as hypotonia. Hypotonia can be caused by nervous system dysfunction or imbalances in certain ions in the bloodstream. Hypotonic muscles have a flaccid appearance and do not function properly. Conversely, excessive muscle tone is referred to as hypertonia. This takes two forms: spasticity, which is a type of stiffness related to uncontrolled reflexes, and rigidity, a stiffness not associated with reflexes.
Learn By Doing 11.3
How can a blood test help diagnose the cause of muscle dysfunction?
Why would some muscle cells be contracted even if the muscle was not producing movement?
- To maintain posture.
- To prevent muscles from becoming weak.
- Both of the above answers.
- Neither of the above answers.
Hint: These contracted muscle fibers provide muscle tone.
Skeletal Muscle Organ Anatomy
Learning Objective
Describe the composition of the connective tissue layers that surround each cell, fascicle, muscle, and group of muscles.
Skeletal muscles, which are the organs of the skeletal muscle system, include three layers of connective tissue that enclose and provide structure to the muscle as a whole. The outer layer of each muscle fiber is wrapped with a layer of connective tissue called the epimysium (“epi-” means “outside” or “over,” and “-mysium” refers to “muscle”). The epimysium allows muscle to contract and move powerfully while maintaining its structural integrity; it also separates muscle from other tissues and organs. It is composed of a thick layer of collagen fibers. The epimysium is surrounded by fascia, which is a type of connective tissue that is found around body organs. Deep fascia surrounds groups of muscles, sometimes joining with tendons to strengthen the bone attachment, whereas superficial fascia lies between muscle and skin. Some fascia contain adipose tissue that insulates and protects muscle.
Inside each skeletal muscle, muscle fibers (a single muscle cell is called a muscle fiber; not to be confused with fibers found in connective tissue) are organized into groups called fascicles, or bundles of muscle fibers (cells). Some of these fascicles can be seen without a microscope when a muscle is cut open. Each fascicle contains many long muscle fibers bound by a middle layer of connective tissue called the perimysium (“peri-” means “around”). Similar to the epimysium, perimysium contains collagen fibers, but perimysium also contains elastic fibers.
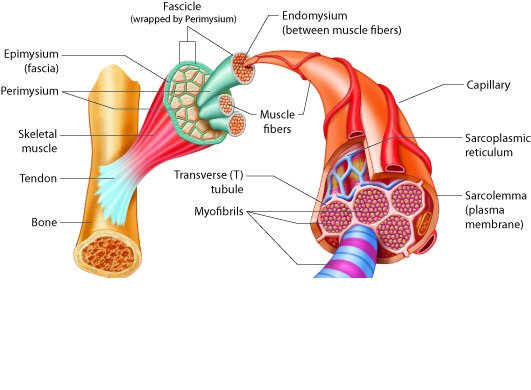
Inside each fascicle, each individual muscle fiber is encased in a connective tissue layer called the endomysium (“endo-” means “inside”). The endomysium forms a thinner layer than the dense epimysium and perimysium and contains areolar and reticular tissues which form loose, delicate networks. The endomysium of muscle fibers connect to each other to form a loose complex within a fascicle. Small blood vessels and motor neurons pass through the endomysium to support and activate each muscle fiber.
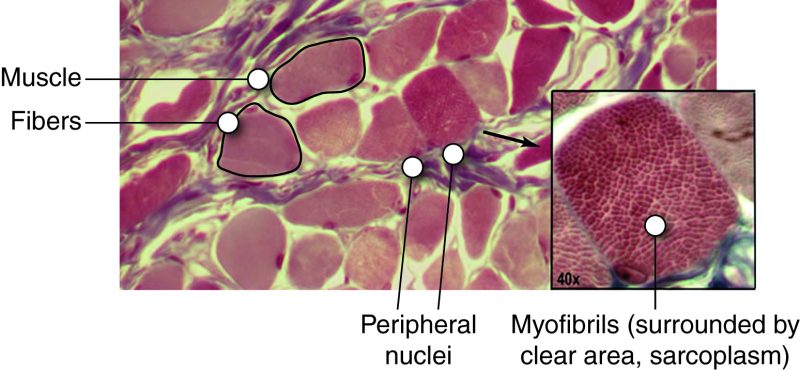
The sarcolemma or plasma membrane of a skeletal muscle fiber is located just under the endomysium. The sarcolemma is the site of action potential conduction, which triggers muscle contraction. Within the sarcolemma is the sarcoplasm, the cytoplasm of the muscle cell.
Learn By Doing 11.4
Imagine that you are buying a bulk package of cookies such as the one shown below.
There is one big box that is your entire muscle. Inside that box are four smaller boxes that are your fascicles. Inside each of these smaller boxes are four rolls of cookies. Use this analogy to answer the following questions about muscle organization.
Hint: “endo-” means “inside.” “peri-” means around. “epi-” means “outside” or “over.”
The entire package (the muscle) is surrounded by the Outer Box called:
- Epimysium
- Perimysium
- Ecomysium
- Endomysium
The entire package (the muscle) is then divided into smaller units (the fascicles) that are surrounded by their own boxes called:
- Epimysium
- Perimysium
- Ecomysium
- Endomysium
The smaller units (the fascicles) are divided into rolls of cookies (individual cell) surrounded by plastic wrap called:
- Epimysium
- Perimysium
- Ecomysium
- Endomysium
Skeletal Muscle Movement
Learning Objective
Define the terms: aponeuroses, tendons, and bursae.
Skeletal muscles, which are the organs of the skeletal muscle system, generally act by pulling on bones to produce movement. To pull the bone, muscles need to attach to the bone, either directly or indirectly. The epimysium of a muscle can attach directly to bone or cartilage to form a direct attachment. An indirect attachment is formed when the connective tissue layers, the epimysium, perimysium, and endomysium form a complex at the end of the muscle.
For connections, muscles require either a tendon or a broader sheet of connective tissue called an aponeurosis. Muscles connect to muscles via aponeuroses, and muscles attach to bones via tendons or aponeuroses. Thus, when a muscle contracts, the force of movement is transmitted through the attachment, which pulls on the bone to produce skeletal movement.
Tendons also help to stabilize the joints. Tendons are a common form of attachment because the collagen fibers are more resistant to tearing than direct muscle tissue attachment to bone would be, and the compact form of the tendon requires a small amount of space. Tendons can be easily seen as the hand is flexed, causing the thick, cordlike tendons of the forearm to stand out prominently. The calcaneal (Achilles) tendon is visible from the heel to the calf. Some tendons are also surrounded by a connective tissue layer called a tendon sheath, which protects the tendon as it moves. Fluid-filled sacs called bursae also join to tendons to reduce friction as the tendon moves. Bursae are present in connective tissue near bone, where tendons experience friction, but they occasionally arise in other areas due to stress caused by movement.
Example: Bursitis
Keep in mind that the range of motion produced by muscles is restricted by the anatomy of the bones and other support structures involved in a particular joint. Consider the movements of the hip and shoulder joints, both of which are freely moveable. The hip is the attachment point for the thigh and leg. The shoulder is the attachment point for the arm and forearm. The shoulder can produce movements that the hip cannot because of the anatomy of the bones involved and the ligaments (connective tissue) associated with the joint. Hold your arm out to the side and move your thumb around the axis of the arm in a 360-degree circle. Can you do that with your hip and leg? No, you can’t. There are some people who appear to be “double-jointed”; they are able to push their joints past the normal limits of movement. The reason they can do this is because the ligaments of their joints are “loose” compared with someone who is not “double-jointed.” This ability appears to have a genetic basis.
For muscles attached to the bones of the skeleton, the location of the connection determines the force, speed, and type of movement. These characteristics depend on each other and can explain the general organization of the muscular and skeletal systems.
Learn By Doing 11.5
A jogger may experience more bursitis in their knees, but a pitcher may experience more bursitis in their shoulder. Which of the following would explain this difference?
- Joggers have a lot of mechanical stress on their knees and pitchers have a lot of mechanical stress on their shoulders.
- Joggers have a lot of mechanical stress on their shoulders and pitchers have a lot of mechanical stress on their knees.
- There should not be a difference in where bursitis occurs.
- This infection occurs at joints that do not have a lot of stress placed on them.
Hint: -itis indicates “inflammation” and can be caused by stress.
Muscle Movement and Gross Anatomy of Muscles
Learning Objectives
- Define the terms prime mover (or agonist), antagonist, and synergist.
- Identify the conventions for naming muscles.
Skeletal muscles are arranged in pairs for balance and to work efficiently.
Some muscles function as agonists or prime movers. An antagonist is a muscle that opposes the action of the agonist. When an agonist contracts to produce a movement, the corresponding antagonist will be stretched and contract with sufficient tension to control the movement. For instance, when your biceps muscle acts to flex your forearm, the triceps muscle contracts slightly to prevent you from flexing your forearm too quickly or too strongly.
Agonist and antagonist muscles work against one another to control and balance movements. Other muscles, called synergists (from synergy, to work together), improve the efficiency of an agonist muscle. Synergists may provide additional pull or stabilization, and their assistance to a particular movement may change throughout the progression of the movement.
This table provides examples of agonist-antagonist combinations.
Agonist | Antagonist | Movement |
Biceps brachii—located on the anterior surface of the upper arm | Triceps brachii—located on the posterior surface of the upper arm | The biceps brachii flexes the forearm while the triceps brachii extends it. |
Hamstrings—a group of three muscles located on the posterior of the thigh | Quadriceps femoris—a group of four muscles located on the anterior of the thigh | The hamstrings flex the lower leg. The quadriceps femoris extend the lower leg. |
The naming of skeletal muscles is based on numerous criteria including the location of the muscle, the shape, the orientation, the number of the muscle in a group, the action it generates, and the location of its origin(s) and insertion(s).
Location: Some muscle names indicate the bones or body region with which the muscle is associated. For example, the frontalis muscle is located on top of the frontal bone of the skull. The rectus femoris (femur) and brachioradialis (arm and radius) are also good examples.
Shapes: Muscles with distinctive shapes are often named for the shape. The trapezius in the neck and back is similar to a trapezoid. You have already learned about muscles that have “orbicularis” in the name. The size of the muscle is the source of the names of the muscles of the buttocks: gluteus maximus (largest), gluteus medius (medium), and gluteus minimus (smallest). There are also muscles with names that contain brevis (short), longus (long), lateralis (lateral side or away from the midline), and medialis (toward the midline). Some practice with the names of common muscles will help you with the descriptions that indicate muscle size or location.
Orientation: The direction of the muscle fibers and fascicles relative to the midline are also used to describe muscles, such as the rectus (straight) abdominis or the internal and external oblique (at an angle) muscles of the abdomen.
Numbers in a group: Some muscles are named according to the number of muscles in a “group.” One example of this is the quadriceps, a group of four muscles located on the anterior (front) of the thigh. Other muscle names can give you a clue as to how many origins a particular muscle has, such as the biceps brachii or triceps brachii. Bi- indicates that the muscle has two origins and tri- indicates three.
Action: When muscles are named for the movement they produce, you will find action words in their name. Some examples are flexor (decreases the angle at the joint), extensor (increases the angle at the joint), abductor (moves the bone away from the midline), or adductor (moves the bone toward the midline).
Attachments: The location of a muscle’s orientation and insertion can also be used in its name. The origin is the unmoving, stable connection of the muscle to a structure, usually a bone. The insertion is the moveable end of the muscle, and it inserts onto various structures in the body. For muscles named this way, the origin is always named first. For instance, the sternocleidomastoid muscle of the neck has a dual origin on the sternum (sterno) and clavicle (cleido), and it inserts on the mastoid process of the temporal bone.
The following table lists important muscle terminology.
Name | Definition | Example |
Direction Relative to the Midline of the Body | ||
rectus | straight | rectus abdominis |
transverse | at right angle | transverse abdominis |
oblique | diagonal | external oblique |
Relative Size | ||
maximus | largest | gluteus maximus |
medius | medium | gluteus medius |
minimus | smallest | gluteus minimus |
longus | long | longus capitis |
brevis | short | extensor carpi radialis brevis |
latissimus | widest | latissimus dorsi |
longissimus | longest | longissimus thoracis |
magnus | large | adductor magnus |
major | larger | rhomboid major |
minor | smaller | rhomboid minor |
vastus | huge | vastus medialis |
Relative Shape | ||
deltoid | triangular | deltoid |
trapezius | trapezoidal | trapezius |
serratus | serrated | serratus anterior |
rhomboid | diamond-shaped | rhomboid minor |
orbicularis | circular | orbicularis oris |
pectinate | comb-like | pectineus |
piriformis | pear-shaped | piriformis |
platys | flat | platysma |
quadratus | four-sided and square | quadratus lumborum |
gracilis | slender | gracilis |
Action | ||
flexor | decreases the angle at a joint | flexor digitorum superficialis |
extensor | increases the angle at a joint | extensor digitorum |
abductor | moves the bone away from the midline | abductor pollicis longus |
adductor | moves the bone toward the midline | adductor magnus |
levator | elevates a body part | levator scapulae |
depressor | lowers a body part | depressor labii inferioris |
supinator | turns the palm anteriorly | supinator |
pronator | turns the palm posteriorly | pronator teres |
sphincter | decreases the size of an opening | external anal sphincter |
tensor | tenses a body part | tensor fasciae latae |
rotator | rotates a bone around its longitudinal axis | rotator |
Number of Origins | ||
biceps | two | biceps brachii |
triceps | three | triceps brachii |
quadriceps | four | quadriceps femoris |
Did I Get This? 11.1
Which of the following is not a function of a synergist?
- It helps the agonist work more efficiently
- It prevents the movement of another joint
- It stabilizes the origin of the agonist
- It stabilizes the insertion of the agonist
Which of the following is not a method of naming muscles?
- Size
- Location
- Agonist
- Shape
Muscular Levels of Organization
The force generation required for skeletal muscle function occurs at the molecular level. You can develop a better understanding of the properties of cells and tissues by studying the molecular mechanisms common to the cells involved:
- Molecular level— actin and myosin
- Microscopic level— sarcomere, specialized organelles, myofibrils
- Cellular level— myoblasts and myofibers
- Tissue level— neuromuscular junctions and fascicles
- Organ level— major skeletal muscles of the body
Much of the unit on muscle will include movements of ions for signaling. Complete the exercise below to ensure that you can identify their symbols.
Learn By Doing 11.6
Correctly identify the ions K+, Na+, Ca2+, Cl–.
- chloride
- sodium
- calcium
- potassium
Molecular Level– Molecules of the Myofilaments
Learning Objectives
- Describe the interaction of actin and myosin in force generation.
- Identify which cofactors (ions and proteins) regulate actin-myosin force generation.
- Predict the effect of decreased ATP on cross-bridges and muscle contraction.
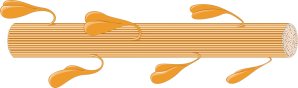
Myosin is a protein that converts the chemical energy stored in the bonds of ATP into the kinetic energy of movement. Myosin is the force-generating protein in all muscle cells, and a coordinated effort among many myosin molecules pulling on actin generates force for movement. Myosin molecules have two main structural parts. The tail of a myosin molecule consists of two polypeptide subunits wound together, whereas the head is composed of two globular subunits. Each club-like head has a binding site for actin. Each pair of myosin heads also has a binding site for ATP. This area of myosin is referred to as an ATPase since it is an enzyme that hydrolyzes ATP to ADP and inorganic phosphate (Pi). The tail of a myosin molecule connects with other myosin molecules to form the central region of a thick filament. In contrast, the heads align on either end of the thick filament where thin filaments overlap with the thick filament. The point at which the head and tail of the molecule meet is flexible and allows the head to move back and forth. This allows myosin to pull on the thin filament when the two filaments bind during muscle contraction.

(Created by Servier Medical Art and used under a Creative Commons Attribution 3.0 Unported License.)
Thin filaments are made of individual globular (spherical) protein subunits (G-actin) that assemble linearly into two helical (twisted) filaments along with several regulatory proteins. Each G-actin has an active site that, if uncovered, can bind myosin. In skeletal muscle. Tropomyosin and troponin regulate contraction by controlling the interaction between actin and myosin filaments. Tropomyosin blocks myosin binding sites on the actin filament, preventing the formation of structures called cross-bridges and blocking muscle contraction. Calcium ions trigger muscle contraction by binding to troponin. Troponin alters its shape; this drags tropomyosin off the myosin binding sites on actin, allowing binding between actin and myosin. Muscle contraction occurs. Calcium is generally an important molecule in muscle function, as we will discuss later.
The Cross-bridge Cycle and ATP
ATP binding to myosin causes it to release actin, allowing actin and myosin to detach from each other. After this occurs, ATP is converted to ADP and Pi. The energy released during ATP hydrolysis changes the angle of the myosin head into a “cocked” position. The myosin head is then in a position for further movement, possessing potential energy; however, ADP and Pi are still attached. Cross-bridges between the actin and myosin form if the active sites on actin are exposed, as myosin binds to actin while ADP and Pi are still bound to it. Pi is then released, causing myosin to form a stronger attachment to actin, and the myosin head moves toward the middle of the sarcomere (the M line), pulling actin along with it. As actin is pulled, the thin filament moves approximately 10 nanometers toward the M line. This movement is called the power stroke, as the thin filament “slides” over the myosin. ADP is released during this step. If, however, actin binding sites are covered and unavailable, the myosin will remain in the high energy configuration with ATP hydrolyzed but still attached.
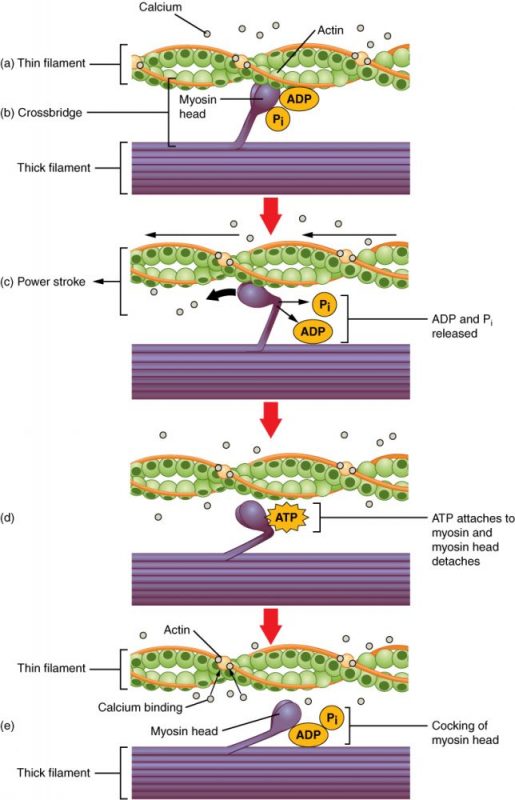
When the myosin head is “cocked,” it contains potential energy and is in a high-energy configuration. This energy is expended as the myosin head moves through the power stroke; at the end of the power stroke, the myosin head is in a low-energy position. After the power stroke, ADP is released. However, the cross-bridge formed is still in place, and actin and myosin are bound together. ATP can then attach to myosin, which allows the cross-bridge cycle to start again and further muscle contraction can occur.
To summarize, for a muscle contraction to occur, tropomyosin must change conformation, uncovering the myosin-binding site on an actin molecule and allowing cross-bridge formation. This can only happen in the presence of calcium, which is kept at extremely low concentrations in the sarcoplasm. If present, calcium ions bind to troponin, causing conformational changes in troponin that allow tropomyosin to move away from the myosin binding sites on actin. Once the tropomyosin is removed, a cross-bridge can form between actin and myosin, triggering contraction. Cross-bridge cycling continues until Ca2+ ions and ATP are no longer available, and tropomyosin again covers the binding sites on actin.
Example:Rigor and Rigor Mortis
At the end of the power stroke, the actin-myosin cross-bridge is still in place (until ATP binds to the myosin head to change its shape). When energy is depleted, ATP is no longer available to bind to myosin; without ATP, actin remains bound to myosin, making both relaxation and further contraction impossible. This state, with an intact cross-bridge and depleted ATP, is called rigor.
This situation is exemplified during rigor mortis, which occurs after death. Dead cells can no longer produce ATP. Without ATP, the myosin heads cannot detach from actin. Recall that ATP is required for the myosin to come off of the actin. Rigor mortis eventually subsides as proteins in the body, including actin and myosin, degrade.
Learn By Doing 11.7
Which protein does not stay bound to actin?
- myosin
- troponin
- tropomyosin
- none of these
Which protein actively moves during muscle contraction?
- myosin
- troponin
- tropomyosin
- actin
Which molecule in the sarcomere uses ATP to generate force?
- Acetylcholine
- Troponin
- Actin
- Myosin
What roles do calcium ions and ATP play in muscle contraction?
Hint: Think about what things might interfere with the cross-bridge cycle and how these occur.
How can medical examiners determine the time of death based on the state of rigor mortis?
Hint: Think about changes during muscle contraction.
Regenerating ATP in Muscle Fibers
In addition to its direct role in the cross-bridge cycle, ATP also provides the energy for the active-transport Na+/K+and Ca2+pumps. Muscle contraction does not occur without sufficient amounts of ATP. The amount of ATP stored in muscle is very low, only sufficient to power a few seconds’ worth of contractions. As it is broken down, ATP must therefore be regenerated and replaced quickly to allow for sustained contraction. There are three mechanisms by which ATP can be regenerated: creatine phosphate metabolism, anaerobic glycolysis, and aerobic respiration.
Creatine phosphate is a phosphagen, which is a compound that can store energy in its phosphate bonds. In a resting muscle, excess ATP (adenosine triphosphate) transfers its energy to creatine, producing ADP (adenosine diphosphate) and creatine phosphate. When the muscle starts to contract and needs energy, creatine phosphate and ADP are converted into ATP and creatine by the enzyme creatine kinase. This reaction occurs very quickly; thus, phosphagen-derived ATP powers the first few seconds of muscle contraction. However, creatine phosphate can only provide approximately 15 seconds’ worth of energy, at which point another energy source has to be available.
After the available ATP from creatine phosphate is depleted, muscles generate ATP using glycolysis. Glycolysis is an anaerobic process that breaks down glucose (sugar) to produce ATP; however, glycolysis cannot generate ATP as quickly as creatine phosphate. The sugar used in glycolysis can be provided by blood glucose or by metabolizing glycogen that is stored in the muscle. Each glucose molecule produces two ATP and two molecules of pyruvate, which can be used in aerobic respiration or converted to lactic acid.
If oxygen is available, pyruvic acid is used in aerobic respiration. However, if oxygen is unavailable, pyruvic acid is converted into lactic acid, which may contribute to muscle fatigue and pain. This occurs during strenuous exercise when high amounts of energy are needed, but oxygen cannot be delivered to muscles at a rate fast enough to meet the whole need. Anaerobic glycolysis cannot be sustained for very long (approximately one minute of muscle activity), but it is useful in facilitating short bursts of high-intensity output. Glycolysis does not utilize glucose very efficiently, producing only two ATP molecules per molecule of glucose, and the by-product lactic acid contributes to muscle fatigue as it accumulates. Lactic acid is transported out of the muscle into the bloodstream, but if this does not happen quickly enough, lactic acid can cause cellular pH levels to drop, affecting enzyme activity and interfering with muscle contraction.
Aerobic respiration is the breakdown of glucose in the presence of oxygen to produce carbon dioxide, water, and ATP. Aerobic respiration in the mitochondria of muscles uses glycogen from muscle stores, blood glucose, pyruvic acid, and fatty acids. Approximately 95 percent of the ATP required for resting or moderately active muscles is provided by aerobic respiration. Aerobic respiration is much more efficient than anaerobic glycolysis, producing approximately 36 ATP molecules per molecule of glucose. However, aerobic respiration does not synthesize ATP as quickly as anaerobic glycolysis, meaning that the power output of muscles declines, but lower-power contractions can be sustained for longer periods.
Phosphorylation of creatine phosphate | Anaerobic glycolysis | Aerobic metabolism | |
Energy source | Creatine phosphate | Glucose | Glucose, pyruvic acid, fatty acids |
Rate of ATP synthesis | Very fast | Fast | Slow |
Number of ATPs produced per unit | 1 | 2 | 36 |
Oxygen required | No | No | Yes |
The table above compares the three ways that ATP can be regenerated. The combination of different processes and varied energy sources is important for different types of muscle activity. As an analogy, a cup of coffee with lots of sugar provides a quick burst of energy but not for very long. A balanced meal with complex carbohydrates, protein, and fats takes longer to impact us but provides sustained energy.
Muscles require a large amount of energy and thus require a constant supply of oxygen and nutrients. Blood vessels enter muscle at its surface, after which they are distributed throughout the entire muscle. Blood vessels and capillaries are found in the connective tissue that surrounds muscle fascicles and fibers, allowing oxygen and nutrients to be supplied to muscle cells and metabolic waste to be removed. Myoglobin, which binds oxygen similarly to hemoglobin and gives muscle its red color, is found in the sarcoplasm.
Example:Exercise and Energy
After the first few seconds of exercise, available ATP is used up. After the next few minutes, cellular glucose and glycogen are depleted. After the next 30 minutes, the body’s supply of glucose and glycogen is depleted. After that time, fatty acids and other energy sources are used to make ATP. That’s why we should exercise for more than 30 minutes to lose weight (i.e. lose fat). Sometimes, time is important.
Learn By Doing 11.8
Athletes will train differently depending on what type of sport they are engaging in. If an athlete was training to become a bodybuilder what would their main source of energy for respiration be?
- Glucose
- Fatty acids
- Phosphate
- Protein
Hint: Bodybuilders use a lot of short, fast bursts of energy.
Microscopic Level—The Sarcomere and Myofilaments
Learning Objectives
- Define the contractile unit of skeletal muscle.
- Describe the organization of a sarcomere in terms of the Z line, M line, thick filament, and thin filament.
- Predict what effect changes in thick and thin filament overlap would have on muscle function.
- Identify the critical components of the sliding filament model of muscle contraction.
The fundamental functional unit of muscle is called a sarcomere. One muscle may contain as many as 100,000 of the repeating sarcomere units. In the sarcomere, the myofilaments (thick filaments and thin filaments) are organized into parallel units. Sarcomeres were first identified by microscopic imaging (histology), and the nomenclature (naming) described below reflects their microscopic “appearance.”
Myofilaments are organized structures in muscle cells that contain the proteins actin and myosin. Thin filaments are formed by a number of proteins. A single thin filament is composed of 300-400 globular actins (G-actin), with 1 nebulin molecule and 40-60 troponin and tropomyosin molecules. Actin filaments are thin, causing the actin “rope” to appear skinny.
Bundles of over 200 myosin proteins form a thick filament. The thick filament myosin heads “walk” along the actin thin filaments. The myosin filament contains many myosins bundled up with all of the head groups sticking out so that it looks “fluffy.” That fluffiness makes it look thick.
Histological sections of muscle show the anatomical features of sarcomeres. Thick filaments, composed of myosin, are visible as the band of a sarcomere. Thin filaments, composed of actin, attach to a protein in the Z disc (or line), and they are present across the entire length of the I band and a portion of the A band. The region where thick and thin filaments overlap has a dense appearance, as there is little space between the filaments. This zone where thin and thick filaments overlap is very important to muscle contraction, as it is the site where filament movement starts. Thin filaments do not extend completely into the A bands, leaving a central region of the A band that only contains thick filaments. This central region of the A band looks slightly lighter than the rest of the A band and is called the H zone. The middle of the H zone has a vertical line called the M line, where accessory proteins hold together thick filaments.
The table below summarizes the microscopic bands of the sarcomere. In this chapter, we will focus primarily on the M- and Z-lines.
Microscopically Visible Feature | Composition |
A band | Region of thick-filament myosin proteins |
H zone | The central region of the A band with no overlapping actin proteins when a muscle is relaxed |
I band | Region of thin-filament actin proteins, with no myosin |
M line | M line accessory proteins in the center of myosin thick filament perpendicular to the sarcomere |
Z disc | Zig-zag line of proteins and actin-binding proteins perpendicular to the sarcomere |
Learn By Doing 11.9
Which protein is found in the thick filaments?
- Troponin
- Actin
- Tropomyosin
- Myosin
Hints: The thick filaments and thin filaments interact in the sarcomere. The thick filaments pull the thin filaments.
Which of the following describes the orientation of both thick and thin filaments in the sarcomere?
- perpendicular
- parallel
- thin and thick filaments are found in different parts of the sarcomere
- skewed
Hint: Which direction would allow the summing of individual actin-myosin cross-bridge forces?
Sliding Filament Model of Contraction
For a muscle cell to contract, the sarcomere must shorten. However, thick and thin filaments—the components of sarcomeres—do not shorten. Instead, they slide by one another, causing the sarcomere to shorten while the filaments remain the same length. The sliding filament theory of muscle contraction was developed to fit the differences observed in the named bands on the sarcomere at different degrees of muscle contraction and relaxation. The mechanism of contraction is the binding of myosin to actin, forming cross-bridges that generate filament movement.
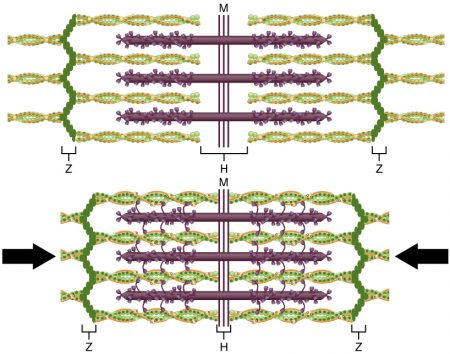
When a sarcomere shortens, some regions shorten whereas others stay the same length. A sarcomere is defined as the distance between two consecutive Z discs ( sometimes called stance between the Z discs is reduced. Thin filaments are pulled by the thick filaments toward the center of the sarcomere until the Z discs approach the thick filaments. The zone of overlap, in which thin filaments and thick filaments occupy the same area, increases as the thin filaments move inward. Thus the thick and thin filaments—the components of sarcomeres—do not shorten. Instead, they slide by one another, causing the sarcomere to shorten while the filaments remain the same length.
To summarize, the striated appearance of skeletal muscle tissue is a result of repeating bands of the proteins actin and myosin that occur along the length of myofibrils. Myofibrils are composed of smaller structures called myofilaments. There are two main types of myofilaments: thick filaments and thin filaments. Thick filaments are composed of the protein myosin. The primary component of thin filaments is the protein actin.
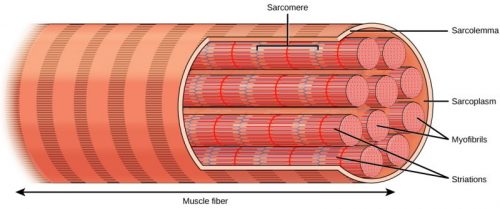
The thick and thin filaments alternate with each other in a structure called a sarcomere. The sarcomere is the unit of contraction in a muscle cell. Contraction is stimulated by an electrochemical signal from a motor neuron associated with the muscle fiber (discussed later in the chapter). For a muscle cell to contract, the sarcomere must shorten. However, thick and thin filaments do not shorten. Instead, they slide by one another, causing the sarcomere to shorten while the filaments remain the same length. The sliding is accomplished when a molecular extension of myosin, called the myosin head, temporarily binds to an actin filament and changes shape, bending and dragging the thin filament toward the center of the sarcomere. The myosin head then releases its actin filament, relaxes, and then repeats the process, dragging the two filaments further past each other. The combined activity of many binding sites and repeated movements within the sarcomere causes it to contract. The coordinated contractions of many sarcomeres in a myofibril lead to the contraction of the entire muscle cell and ultimately the muscle itself. The movement of the myosin head requires ATP, which provides the energy for the contraction.
Learn By Doing 11.10
Which of the following shortens during muscle contraction?
- the thin filament
- the sarcomere
- the thick filament
- actin
- myosin
Describe how actin and myosin, and the thick and thin filaments, are related to the M line and Z line.
Microscopic Level– Organelles
Muscle fibers contain organelles found in all cells, including nuclei, the endoplasmic reticulum, mitochondria, and the Golgi apparatus. The amount and organization of organelles, however, are slightly different in muscle cells. Actin is found inside every cell in the body, but actin is specially organized within the sarcomeres of muscle cells. Muscle cells also have extremely high numbers of mitochondria to produce ATP for force generation. Recall that an ATP molecule is required for one myosin to perform one power stroke.
Organelles and Structures Specific to Skeletal Muscle Cells
Each skeletal muscle fiber is a single cell produced from the fusion of many precursor cells called myoblasts. These fused cells are therefore quite large, with diameters of up to 100 micrometers and lengths of up to 30 centimeters. Compare this with a cell of the skin which is a cube of 20 micrometers in nearly every diameter. In muscle fibers, sarcomeres arrange into parallel structures called myofibrils, so both the Z disc and the M line hold myofilaments in place to maintain the structural arrangement and layering.
There is some special nomenclature associated with these fused cells. Each skeletal muscle fiber cell has more than one nucleus, which is referred to as multinucleate. The plasma membrane of this fused skeletal muscle cell is called the sarcolemma. The muscle interacts with the nerves that stimulate the muscle at the sarcolemma, and we will describe this interaction later. Within the sarcolemma is the sarcoplasm, which is the cytoplasm of the fused muscle fiber. The sarcoplasm has most of the same components as standard cytoplasm in addition to high levels of the protein myoglobin, which stores oxygen.
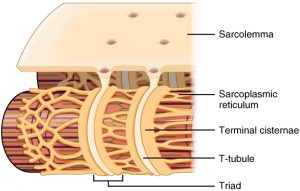
The sarcolemma also contains many structures similar to the plasma membrane of other cells, but it also possesses structures unique to muscle cells. The sarcolemma has transverse tubules, or T tubules, which are indentations of the sarcolemma into the interior of the cell along the length of the muscle cell. The T tubules are filled with extracellular fluid, and they conduct the action potential deep into the interior of the muscle cells, which can be very large. With T-tubules, nerves can stimulate entire muscles and muscle groups quickly and effectively. Without T tubules, action potential conduction into the interior of the cell would happen much more slowly, causing delays between neural stimulation and muscle contraction, resulting in slower, weaker contractions.
Inside skeletal muscle fibers is a network of membranous tubules called the sarcoplasmic reticulum (SR), which is similar to the smooth endoplasmic reticulum found in other cells. SR tubules are filled with high-calcium fluid, and they surround each myofibril. Terminal cisternae are expanded regions of the SR that form on either side of each T tubule extending into the cell. A grouping consisting of a T tubule from the outside of the muscle fiber, and two terminal cisternae, from the inside of the muscle fiber, is called a triad.
T tubules conduct an action potential along the surface of the muscle fiber into triads that trigger the release of Ca2+ions from the nearby terminal cisternae. This, in turn, triggers muscle contraction when the calcium ions in the sarcoplasm can bind to the troponin of the sarcomeres.
The table below summarizes the information on organelles within skeletal muscle fibers.
Name of Cellular Structure | Function |
Sarcolemma | the membrane of the fused skeletal fiber |
Sarcoplasm | the cytoplasm of the fused skeletal fiber |
Sarcoplasmic reticulum | the endoplasmic reticulum of the fused skeletal fiber, storage site of Ca2+ |
Terminal cisternae | enlarged sarcoplasmic reticulum structures store calcium and surround T tubules |
Transverse tubules (T tubules) | tubes of sarcolemma that penetrate into the cell, filled with extracellular fluid, allowing conduction of the muscle action potential deep into the muscle fiber |
Triad | one T tubule and two terminal cisternae |
Did I Get This? 11.2
Below are descriptions that relate to one of the major structures of a skeletal muscle fiber. Match one of the phrases below to the proper part of the muscle fiber. Names may be used more than once.
Myofibrils
Sarcoplasm
Sarcoplasmic reticulum
Sarcolemma
Sarcomere
Triad
Transverse tubule
- Contains 2 Z discs
- The plasma membrane of a fused skeletal muscle cell
- Contains a T tubule and two terminal cisternae
- Contains an M line
- Stores calcium ions (Ca2+)
- An extension of the sarcolemma
- Location of myoglobin
- Site of nerve interaction
- The cytoplasm of a fused skeletal muscle cell
- Similar to smooth endoplasmic reticulum
- Composed of many thick and thin filaments
Which of the following is correct about Ca2+ in skeletal muscle?
- It is stored in the T tubules.
- It is unique to muscle cells.
- It conducts the action potential in the sarcolemma
- It binds to troponin of the sarcomeres.
Why are the T tubules important for coordinating muscle contraction?
- They produce ATP for energy.
- They prevent the release of Ca2+ions.
- They conduct action potentials to the interior of the cell.
- They store oxygen in hemoglobin.
The Cellular Level of Organization
As you have learned, each skeletal muscle fiber is a skeletal muscle cell, also known as a skeletal myocyte (“myo-” refers to “muscle” and “-cyte” refers to “cell”). Within each muscle fiber are myofibrils, long cylindrical structures that lie parallel to the muscle fiber. Myofibrils run the entire length of the muscle fiber. They attach to the plasma membrane, called the sarcolemma, at their ends so that as myofibrils shorten, the entire muscle cell contracts.
Each skeletal muscle fiber is formed from the fusion of myoblast precursor cells. Cell fusion leads to multinucleation of each mature muscle fiber. Each myoblast, the embryonic cell type that differentiates into muscle, contributes one nucleus when the muscle fiber is formed during development.
During development, individual myoblasts (“-blast” refers to “building” … like osteoblasts) migrate to different regions in the body and then fuse to form myotube. A myotube is a type of syncytium, which is the term used for a group of fused cells. Skeletal muscle cells are multinucleate because the syncytium (“syn-” means “same” and “cyt” refers to “cytoplasm”) fusion retains the nucleus of each contributing myoblast. This syncytium leads to the collective sarcoplasm and sarcolemma.
Mature muscle does not grow through this process. Mature cells can change in size, but new cells are not formed when muscles grow. Instead, structural proteins are added to muscle fibers in a process called hypertrophy (hyper, excessive; trophē, nourish). The reverse, when structural proteins are lost, and muscle mass decreases, is called atrophy (a, not; trophē, nourish). Cellular components of muscles can also undergo changes in response to changes in muscle use.
Although the number of muscle cells is set during development, satellite cells help to repair skeletal muscle fibers. Satellite cells are similar to myoblasts in that they are able to divide, fuse and differentiate. These satellite cells are located outside the muscle fibers and are stimulated to grow and fuse with muscle cells by growth factors that are released by muscle fibers under certain forms of stress. Satellite cells can regenerate muscle fibers to a very limited extent, but they primarily help to repair damage in living cells. Satellite cells facilitate the protein synthesis required for repair and growth. If a cell is damaged to a greater extent than can be repaired by satellite cells, the muscle fibers are replaced by scar tissue in a process called fibrosis. Because scar tissue cannot contract, a muscle that has sustained significant damage loses strength and cannot produce the same amount of force or endurance as it could before being damaged.
Learn By Doing 11.11
Why are mature, contracting skeletal muscle cells amitotic (they do not have the ability to divide to produce new muscle cells)?
- Muscle cells have no DNA.
- Muscle cells have no organelles.
- The cellular structures required for division get in the way of the organized sarcomeres.
- Muscle cells take a very long time to form, and the cells have gotten old and stopped dividing by the time they generate force.
Hints: Think about what is necessary inside a cell to divide by mitosis and what is necessary to generate large amounts of force in the sarcomeres. Also, note that skeletal muscle nuclei are “pushed” to the edges of the cell. What is taking up most of the space inside the cell?
During development, what cells fuse to form muscle fibers?
- Myoblasts
- Osteocytes
- Myocytes
- Satellite cells
Which of the following is not true about satellite cells?
- They are not able to divide.
- They can limitedly regenerate muscle fibers.
- They can fuse with muscle cells.
- They can repair damage in living cells.
Neuromuscular Junctions
Learning Objectives
Skeletal muscle cell contraction occurs after a release of calcium ions from internal stores, which is initiated by a neural signal. Each skeletal muscle fiber is controlled by a motor neuron, which conducts signals from the brain or spinal cord to the muscle.
We stimulate skeletal muscle contraction voluntarily. Electrical signals from the brain through the spinal cord travel through the axon of the motor neuron. The axon then branches through the muscle and connects to the individual muscle fibers at the neuromuscular junction. The folded sarcolemma of the muscle fiber that interacts with the neuron is called the motor end-plate; the folded sarcolemma increases surface area contact with receptors. The ends of the branches of the axon are called the synaptic terminals and do not actually contact the motor endplate. A synaptic cleft separates the synaptic terminal from the motor end-plate, but only by a few nanometers.
Communication occurs between a neuron and a skeletal muscle fiber through the neurotransmitter Acetylcholine (ACh). Neural excitation causes the release of ACh molecules from the synaptic terminal into the synaptic cleft, where they can then bind to the appropriate receptors on the motor endplate. The motor end-plate has folds in the sarcolemma, called junctional folds, that create a large surface area for the neurotransmitter to bind to receptors. Generally, there are many folds and invaginations that increase surface area including junctional folds at the motor endplate and the T-tubules throughout the cells.
Did I Get This? 11.3
Which of the following is a neurotransmitter?
- troponin
- acetylcholine
- sodium ions
Which of the following is the correct order of a signal being passed from a neuron to a muscle?
- Motor end plate, synaptic cleft, axon terminal
- Synaptic cleft, motor end plate, axon terminal
- Axon terminal, synaptic cleft, motor end plate
- None of these is in the correct order.
The neurotransmitter acetylcholine (ACh) is released when an action potential travels down the axon of the motor neuron, resulting in altered permeability of the synaptic terminal and an influx of calcium into the neuron. The calcium influx triggers synaptic vesicles, which package neurotransmitters, to bind to the presynaptic membrane and release acetylcholine into the synaptic cleft by regulated exocytosis.
After being released by the synaptic terminal, ACh diffuses across the synaptic cleft to the motor end plate, where it binds with ACh receptors. These receptors are ligand-gated Na+ ion channels that open to allow the passage of Na+ into the cell when activated. Once two molecules of ACh bind to the receptor, the ion channel part of the receptors opens, and Na+ ions cross the membrane into the muscle cell. This reduces the voltage difference between the inside and outside of the cell, which is called depolarization. ACh binds at the motor end plate, and so this depolarization is called an endplate potential. The depolarization spreads along the sarcolemma, creating an action potential as sodium channels adjacent to the initial depolarization site detect the change in voltage and open. The action potential moves across the entire cell and through the transverse tubule network, creating a wave of depolarization. ACh is broken down by the enzyme acetylcholinesterase (AChE) into acetyl and choline. AChE resides in the synaptic cleft, breaking down ACh so that it does not remain bound to ACh receptors, which would cause unwanted extended muscle contraction.
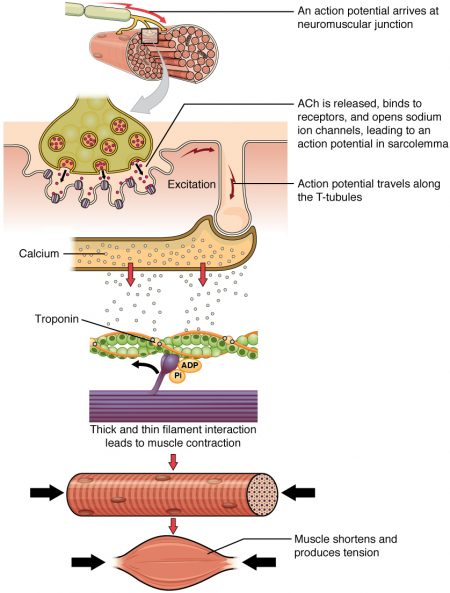
Excitation–Contraction Coupling
Propagation of an action potential and depolarization of the sarcolemma make up the excitation portion of excitation-contraction coupling, the connection of electrical activity and mechanical contraction. The structures responsible for coupling this excitation to contraction are the T tubules and sarcoplasmic reticulum. The critical ion is calcium.
The T tubules are extensions of the sarcolemma and thus carry the action potential along their surface, conducting the wave of depolarization into the interior of the cell. T tubules form triads with the terminal cisternae of the sarcoplasmic reticulum. The terminal cisternae contain high concentrations of Ca2+ ions. As an action potential travels along the T tubule, the nearby terminal cisternae open their voltage-dependent calcium release channels, allowing Ca2+ to flood the sarcoplasm. The influx of Ca2+ increases the amount of calcium available to bind to troponin. Troponin bound to Ca2+ undergoes a shape change that results in tropomyosin moving on the thin filament. When tropomyosin moves, the myosin binding site on the actin is uncovered. This continues as long as excess Ca2+ is available in the sarcoplasm. When there is no more free Ca2+ available to bind to troponin, the contraction will stop. To restore levels back to a resting state, the excess Ca2+ is actively transported back into the sarcoplasmic reticulum.
Learn By Doing 11.12
What would happen to a person who had abnormally high Ca2+ concentration in their sarcoplasm?
- They would not be able to contract their muscles.
- The action potential would not be propagated.
- The troponin would not let the myosin bind.
- They would have unwanted muscle contractions.
Summary of Muscle Contraction at the Cellular Level
The skeletal muscle fiber is excited by large myelinated motor neuron axons whose synaptic terminals form part of the neuromuscular junction. There is one neuromuscular junction for each fiber. What follows is a step-by-step description of the cellular events that lead to muscle contraction.
- An action potential reaches the axon terminals of the motor neuron.
- The action potential activates voltage-gated calcium ion channels in the axon terminal, and calcium rushes in.
- The calcium causes acetylcholine vesicles in the axon terminal to fuse with the membrane, releasing the acetylcholine into the cleft between the axon and the motor end plate of the muscle fiber.
- The acetylcholine diffuses across the cleft and binds to receptors on the motor end plate. Since acetylcholine receptors are also ligand-gated cation channels, sodium rushes in, and potassium rushes out of the muscle fiber. Because the membrane is more permeable to sodium, the muscle fiber membrane becomes more positively charged, triggering an end-plate potential and eventually an action potential.
- The action potentials travel from the surface of the muscle cell along the membrane of T tubules that penetrate into the sarcoplasm of the cell.
- Action potentials along the T tubules cause voltage-dependent calcium release channels in the sarcoplasmic reticulum to open, and Ca2+ions are released from their storage place in the terminal cisternae.
- The Ca2+ binds to the troponin present on the thin filaments of the myofibrils. The troponin changes position, dragging tropomyosin along. Normally the tropomyosin physically obstructs binding sites on actin that allow myosin to form cross-bridges. Once calcium binds to the troponin, the troponin forces the tropomyosin to move out of the way, unblocking the binding sites.
- The myosin head (which is already in a ready state) binds to the newly uncovered binding sites. It then delivers a power stroke, pivoting toward the center of the sarcomere.
- ATP binds the cross-bridge, forcing it to conform in such a way as to break the actin-myosin bond. Another ATP is split to energize the cross bridge again.
Muscle contraction continues as long as Ca2+ is abundant in the sarcoplasm. Throughout this process, the calcium is actively pumped back into the sarcoplasmic reticulum. When no longer present on the thin filament, the tropomyosin changes back to its previous state so as to block the binding sites again. The cross-bridge then ceases binding to the thin filament, and the contractions cease as well. The muscle fiber returns to its pre-contraction length.
The Tissue and Organ Level of Organization– Muscle Twitches and Motor Units
You have already learned about how the information from a neuron ultimately leads to a muscle cell contraction. One action potential in a motor neuron produces one contraction. This contraction is called a twitch. We think of “muscle twitches” as spasms that we can’t control, but in physiology, a twitch is a technical term describing a muscle response to stimulation. A single twitch does not produce any significant muscle contraction. Multiple action potentials (repeated stimulation) are needed to produce a muscle contraction that can produce work.
A twitch can last from a few milliseconds up to 100 milliseconds, depending on the muscle type. The tension produced by a single twitch can be measured by a myogram, which produces a graph illustrating the amount of tension produced over time (see part a of the figure below).
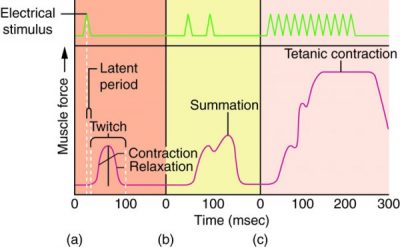
When combined with a plot of electrical signaling, the myogram shows three phases that each twitch undergoes. The first period is the latent period, during which the action potential is propagated along the membrane, and Ca2+ ions are released from the sarcoplasmic reticulum. No tension or contraction is produced at this point, but the conditions for contraction are being established. This is the phase during which excitation and contraction are being coupled, but contraction has yet to occur. The contraction phase occurs after the latent period when cross-bridge formation occurs. This period lasts from the beginning of contraction to the point of peak tension. The last phase is the relaxation phase when tension decreases as contraction stops. Calcium is pumped out of the sarcoplasm, back into the sarcoplasmic reticulum, and cross-bridge cycling stops. The muscle returns to a resting state. There is a very short refractory period after the relaxation phase.
A single twitch does not produce any significant muscle activity in a living body. Normal muscle contraction is more sustained, and it can be modified to produce varying amounts of force. This is called a graded muscle response. The tension produced in a skeletal muscle is a function of both the frequency of neural stimulation and the number of motor neurons involved.
The rate at which a motor neuron delivers action potentials affects the contraction produced in a muscle cell. If a muscle cell is stimulated while a previous twitch is still occurring, the second twitch will not have the same strength as the first; it will be stronger. This effect is called summation or wave summation because the effects of successive neural stimuli are summed or added together (see part b of the figure above). This occurs because the second stimulus releases more Ca2+ ions, which become available while the muscle is still contracting from the first stimulus (the first wave of calcium ions released). This allows for more cross-bridge formation and greater contraction. Because the second stimulus has to arrive before the first twitch has been completed, the frequency of the stimulus determines whether summation occurs or not.
If the frequency of stimulation increases to the point at which each successive stimulus sums with the force generated from the previous stimulus, muscle tension continues to rise until the tension generated reaches a peak point. The tension at this point is about three to four times higher than the tension of a single twitch; this is referred to as incomplete tetanus. Tetanus is defined as a continuous fused contraction (see part c of the figure above). During incomplete tetanus, the muscle goes through quick cycles of contraction with a short relaxation phase. If the stimulus frequency is so high that the relaxation phase disappears completely, contractions become continuous in a process called complete tetanus. This occurs when Ca2+ concentrations in the sarcoplasm reach a point at which contractions can continue uninterrupted. This contraction continues until the muscle fatigues and can no longer produce tension.
This type of tetanus is not the same as the disease of the same name which is distinguished by severe sustained contraction of skeletal muscles. The disease, which can be fatal if left untreated, is caused by the bacterium Clostridium tetani, which is present in most environments. The toxin from the bacterium affects how motor neurons communicate and control muscle contractions, resulting in muscle spasms or sustained contractions, also known as “lockjaw.”
The strength of contractions is controlled not only by the frequency of stimuli but also by the number of motor units involved in a contraction. A motor unit is defined as a single motor neuron and the corresponding muscle fibers it controls. Increasing the frequency of neural stimulation can increase the tension produced by a single motor unit, but this can only produce a limited amount of tension in a skeletal muscle. To produce more tension in an entire skeletal muscle, the number of motor units involved in contraction must be increased. This process is called recruitment.
The size of motor units varies with the size of the muscle. Small muscles contain smaller motor units and are most useful for fine motor movements. Larger muscles tend to have larger motor units because they are generally not involved in fine control. Even within a muscle, motor units vary in size. Generally, when a muscle contracts, small motor units will be the first ones recruited in a muscle, with larger motor units added as more force is needed.
All of the motor units in a muscle can be active simultaneously, producing a very powerful contraction. This cannot last for very long because of the energy requirements of muscle contraction. To prevent complete muscle fatigue, typically motor units in a given muscle are not all simultaneously active, but instead, some motor units rest while others are active, allowing for longer muscle contractions by the muscle as a whole.
Learn By Doing 11.13
Stimulation of additional motor units will increase the strength of contraction. This process is called:
- tetanus
- summation
- contraction
- recruitment
Explain the difference between a muscle twitch and a muscle spasm.
Homeostasis in the Muscular System
Learning Objectives
- Describe how an improperly functioning skeletal muscular system would affect other systems.
- Provide examples of factors that can affect muscle size.
- Explain the role of the muscular system in maintaining temperature homeostasis.
Body Temperature
Skeletal muscles contribute to maintaining temperature homeostasis in the body by generating heat. Muscle contraction requires energy and produces heat as a byproduct of metabolism. All types of muscle produce heat, but because of the large amount of skeletal muscle present in the body, skeletal muscle contributes most greatly to heat production. This is very noticeable during exercise when sustained muscle movement causes body temperature to rise. In cases of extreme cold, shivering produces random skeletal muscle contractions to generate heat as part of the negative feedback mechanism of maintaining body temperature.
Example: Malignant Hyperthermia
Growth of Muscle
Physical training alters the appearance of skeletal muscles and can produce changes in muscle performance. Conversely, a lack of use can result in decreased performance and muscle appearance. As you learned earlier, mature muscle cells grow from hypertrophy, not cell division. The loss of structural proteins and muscle mass occurs during atrophy. Cellular components of muscles can also undergo changes in response to changes in muscle use.
Example: Sarcopenia
Example: Anabolic Steroids
Muscle and Blood Flow
Slow fibers are predominantly used in endurance exercises that require little force but involve numerous repetitions. The aerobic metabolism used by slow-twitch fibers allows them to maintain contractions over long periods. Endurance training modifies these slow fibers to make them even more efficient by producing more mitochondria to enable more aerobic metabolism and more ATP production. Endurance exercise can also increase the amount of myoglobin in a cell, as increased aerobic respiration increases the need for oxygen. Myoglobin is found in the sarcoplasm and stores oxygen. Endurance training can also trigger the formation of more extensive capillary networks around the fiber, a process called angiogenesis, to supply oxygen and remove metabolic waste. To allow these capillary networks to supply the deep portions of the muscle, muscle mass does not greatly increase, maintaining a shorter distance for the diffusion of nutrients and gases. All of these cellular changes result in the ability to sustain low levels of muscle contraction for greater periods of time without fatiguing.
Example: EPO
As discussed previously, there are a number of proteins that are important in regulating and carrying out the contractile process. As you’ve learned, the instructions for how to build proteins are found in each species’ DNA. So it is not a surprise that there are genetic disorders that lead to altered protein formation and dysfunction of the muscular system. Such dysfunction tends to affect many body systems, of which the respiratory system may be the most important. Without the ability to appropriately contract the skeletal muscles of respiration, people cannot live very long. The skeletal muscular system is also very dependent upon a constant supply of ATP. Like many other systems, this requires the intracellular pathways to convert glucose into energy.
Learn By Doing 11.14
Negative feedback occurs when environmental conditions move the body away from optimal conditions. For example, as your body cools below 98.6°F (37°C), the control center in your brain signals that actions need to be taken to raise your body temperature. The muscular system will begin to act. The smooth muscle of the blood vessels in the extremities will constrict and shut the blood to the important organs. What phenomena does this explain:
- One reason that frostbite occurs on the fingers and nose first.
- The feeling of hyperthermia in your extremities.
- The occurrence of varicose veins in the legs.
- The excessive thirst you feel when you are hot.
Hint: You can live without your fingers. Cellular respiration is an aerobic process, and the equation is normally written as C6H12O6 + 6O2 yields 6CO2 + 6H2O and ATP.
Why will people die if their body temperature increases too much?
- The body will undergo spontaneous combustion.
- The body’s metabolic reactions are interrupted.
- The body’s metabolic reactions will begin to go too fast.
- The body will not be able to get enough calcium.
In older people, the ratio of muscle fibers to connective tissue decreases. This may result in
- Less powerful movements
- More powerful movements
- More motor units being activated
- An increase in muscle tension
Endurance training can cause angiogenesis which is an example of how structure and function are related because:
- There is an increase in adipose tissue that increases the endurance of the muscle.
- There is an increase in capillaries that decreases the endurance of the muscles.
- There is an increase in muscle fibers that increases the atrophy of the muscle.
- There is an increase in capillaries that increases the endurance of the muscles.
Integration of Systems
Learning Objectives
- Describe how an improperly functioning skeletal muscular system would affect other systems.
- Describe how muscle tissue within the cardiovascular system contributes to proper function.
- Describe how muscle tissue within the digestive system contributes to proper function.
Think of all of the activities you do that require the functioning of your muscular system. These include walking, chewing, swallowing, breathing, talking, etc. Dysfunctions of this system (such as weakness or hypertonia) can prevent the body from carrying out any of these activities normally. This in turn can affect many other systems. For example, if we can’t bring in appropriate nutrients and oxygen, every other system in the body would be affected, as they are all dependent on fuel sources. Muscles require a large amount of energy and thus require a constant supply of oxygen and nutrients. Blood vessels enter muscle at its surface, after which they are distributed throughout the entire muscle. Blood vessels are found in the connective tissue that surrounds muscles, allowing oxygen and nutrients to be supplied to muscles and metabolic waste to be removed. The epimysium houses the arteries and veins that allow blood to enter and exit muscle. The blood vessels then branch into the epimysium to serve each fascicle and then further branch into the endomysium to form a capillary network that contacts each muscle fiber. The flexible, extensively branched capillary networks are able to withstand muscle contractions without being damaged. Skeletal muscles are under neural control, which means that nerves must be present for the muscle to function. The epimysium contains nerve fibers that branch through the perimysium and epimysium to connect neurons to individual muscle fibers.
Cardiac and Smooth Muscles
Learn By Doing 11.15
Which of the following depends on the proper function of skeletal muscles?
- Walking
- Talking
- Blood flow
- All of the above
Varicose veins occur when valves are not functioning properly and blood that should be flowing toward the heart pools in the vein. Varicose veins are more common in the legs. Which of the following is the best explanation for this?
- Varicose veins are more common in the legs because there are larger veins.
- Varicose veins are more common in the legs because there is more blood in the legs.
- Varicose veins are more common in the legs.
- Varicose veins are more common in the legs because there are more valves in the legs.
Hint: Think about where in your body gravity will pull the blood down the most.
Which of the following is not matched with its proper function in the cardiovascular system:
- Cardiac muscle- contractions of the heart pump blood to the body.
- Smooth muscle- contraction and relaxation allow the shunting of blood to where it is needed.
- Skeletal muscle- contractions around major arteries help move blood to the body.
Which of the following is accomplished using smooth muscle?
- Chewing food
- Mixing food
- Biting food
- Voluntary control of the anal sphincter
*
“Learn By Doing” and “Did I Get This?” Feedback
Learn By Doing 11.1
Let’s test your knowledge of some common facts and myths about the muscular system.
If you eat a lot of protein, you will store it in your muscles, and they will get bigger.
- Fact
No, there is no place in the body that stores excess protein. Excess protein is converted and stored as fat. The only way to make your muscles larger is to exercise. - Myth
Yes, this is a myth. Eating a lot of protein will only cause your liver to convert it into fat for storage.
It takes more muscles to frown than to smile
- Fact
No: It is commonly stated that it takes 43 muscles to frown and 17 to smile but there aren’t even that many muscles in the face! A serious frown and scowl will require more muscles than a smile, but not many people walk around like that! - Myth
Yes, this is a myth. There are 53 facial muscles, and according to David H. Song, MD, FACS, plastic surgeon and assistant professor at the University of Chicago Hospital, 12 of those muscles are involved with smiling and 11 for frowning. Part of the reason for this myth comes from people defining frowns in different ways. However, smiles are contagious – ever see someone smile? We typically imitate it!
The muscle (based on weight) that can generate the most pressure in the human body is the tongue.
- Fact
No, this is a myth. The tongue is the strongest and most versatile muscle in the human body but not the one that can generate the most pressure. - Myth
Yes: The masseter (chewing) muscle is the strongest muscle and produces the greatest pressure for its size. It is estimated that the masseter can generate 200 pounds of pressure!
The hardest working muscle in the body is the heart.
- Fact
Yes, this is a fact. The cardiac muscle of the heart contracts 100,000 times every day! - Myth
No: The heart is really the hardest working muscle; it never gets a chance to rest and pumps 1000 gallons of blood every day!
Skeletal muscle is 75% water.
- Fact
Yes, this is a fact. Water is a critical component of many tissues in the human body; skeletal muscle is one of them. Dehydration can cause muscle fatigue and cramps. - Myth
No: Water is extremely important for the normal functioning of the human body. Skeletal muscle is just one of several tissues that contain a lot of water.
Learn By Doing 11.2
All muscle tissue is formed from muscle fibers and is striated.
- True
While all muscle tissue (smooth, cardiac, and skeletal) is composed of muscle fibers (muscle cells) only skeletal and cardiac muscle cells have a striated appearance. - False
Yes: All muscle tissue (smooth, cardiac, and skeletal) is composed of muscle fibers (muscle cells), but smooth muscle is not striated; skeletal and cardiac are striated.
Which ion is necessary for muscle contraction?
- acetylcholine
No, acetylcholine is a neurotransmitter, not an ion. - potassium
No, while potassium is important in maintaining the voltage in cells it is not the ion that triggers muscle contraction. - calcium
Yes: Calcium is vital for muscle contraction; it is responsible for allowing the muscle to shorten. - myosin
No, myosin is a muscle protein, not an ion.
Learn By Doing #3
How can a blood test help diagnose the cause of muscle dysfunction?
Our Answer: Measuring levels of free ions (calcium, sodium, and potassium) in the blood may suggest why muscle is malfunctioning. However, the cause of the ion imbalance is most likely other aspects of whole-body homeostasis, including issues with the kidneys, skeletal system, or digestive system.
Why would some muscle cells be contracted even if the muscle was not producing movement?
- To maintain posture.
Partially correct: This is only one reason that some muscles would rest and some would stay contracted. - To prevent muscles from becoming weak.
Partially correct: This is only one reason that some muscles would rest and some would stay contracted. - Both of the above answers.
Correct. Muscle fibers must be continually contracting for proper posture. Additionally, it takes longer to activate a muscle if all of the fibers are relaxed. - Neither of the above answers.
No, both prevention of muscle weakness and posture maintenance are reasons for partial muscle contraction.
Learn By Doing 11.4
Imagine that you are buying a bulk package of cookies such as the one shown below.
There is one big box that is your entire muscle. Inside that box are four smaller boxes that are your fascicles. Inside each of these smaller boxes are four rolls of cookies. Use this analogy to answer the following questions about muscle organization.
The entire package (the muscle) is surrounded by the Outer Box called:
- Epimysium
Correct. The epimysium surrounds the entire muscle. - Perimysium
Incorrect. The perimysium surrounds the fascicle. - Ecomysium
Incorrect. This is not a membrane that surrounds the muscle. - Endomysium
Incorrect. The endomysium surrounds the individual muscle cell.
The entire package (the muscle) is then divided into smaller units (the fascicles) that are surrounded by their own boxes called:
- Epimysium
Incorrect. The epimysium surrounds the entire muscle. - Endomysium
Incorrect. The endomysium surrounds the individual muscle cell. - Perimysium
Correct. The perimysium surrounds the fascicle. - Ecomysium
Incorrect. This is not a membrane that surrounds the muscle.
The smaller units (the fascicles) are divided into rolls of cookies (individual cells) surrounded by plastic wrap called:
- Endomysium
Correct. The endomysium surrounds the individual muscle cell. - Perimysium
Incorrect. The perimysium surrounds the fascicle. - Epimysium
Incorrect. The epimysium surrounds the entire muscle. - Ecomysium
Incorrect. This is not a membrane that surrounds the muscle.
Learn By Doing 11.5
A jogger may experience more bursitis in their knees, but a pitcher may experience more bursitis in their shoulder. Which of the following would explain this difference?
- Joggers have a lot of mechanical stress on their knees and pitchers have a lot of mechanical stress on their shoulders.
Correct. Your knees are under a lot of mechanical stress as a jogger, and as a pitcher, your shoulder is under a lot of stress. This mechanical stress can cause inflammation of the bursae. - Joggers have a lot of mechanical stress on their shoulders and pitchers have a lot of mechanical stress on their knees.
Incorrect. Joggers have a lot of mechanical stress on their knees. - There should not be a difference in where bursitis occurs.
Incorrect. Bursitis occurs at joints that are under a lot of mechanical stress. - This infection occurs at joints that do not have a lot of stress placed on them.
Incorrect. Bursitis is an inflammation not necessarily an infection.
Did I Get This? 11.1
Which of the following is not a function of a synergist?
- It helps the agonist work more efficiently
Incorrect. Helping the agonist work more efficiently is one of the functions of a synergist. - Prevents movement of another joint
Incorrect. A synergist will prevent the movement of a joint that is the origin of the agonist and will stabilize this joint. - Stabilize the origin of the agonist
Incorrect. A synergist will prevent the movement of a joint that is the origin of the agonist and will stabilize this joint. - Stabilize the insertion of the agonist
Correct. A synergist will stabilize the origin, not the insertion.
Which of the following is not a method of naming muscles?
- Size
Incorrect. Muscles are named for their size. One example is the gluteus maximus. - Location
Incorrect. Muscles are named for their location. One example is the brachioradialis. - Agonist
Correct. Muscles are not named after their agonist. - Shape
Incorrect. Muscles are named for their shape. One example is the deltoid.
Learn By Doing 11.6
Correctly identify the following ions:
K+ potassium
Na+ sodium
Ca2+ calcium
Learn By Doing 11.7
Which protein does not stay bound to actin?
- myosin
Correct. Myosin only transiently binds to actin during muscle contraction. - troponin
Incorrect. Troponin binds to tropomyosin and actin to regulate actin and myosin interactions. - tropomyosin
Incorrect. Tropomyosin binds parallel to actin to stabilize the filaments. - none of these
Incorrect. These proteins are all actin-binding proteins, but one of them only binds occasionally.
Which protein actively moves during muscle contraction?
- myosin
Correct. Myosin molecules have hinge areas that are activated by ATP hydrolysis. During the power stroke, these hinges return to their resting position. Since actin is bound to myosin by cross-bridges, the thin filament is pulled toward the middle dragging the Z lines along. - troponin
Incorrect. Troponin binds to tropomyosin and actin to regulate actin and myosin interactions. - tropomyosin
Incorrect. Tropomyosin binds parallel to actin to stabilize the thin filaments and covers the active site on actin, preventing muscle contraction. - actin
Incorrect. Actin, and the thin filament, are passively drug toward the middle.
Which molecule in the sarcomere uses ATP to generate force?
- Acetylcholine
Incorrect. Acetylcholine transmits signals from neurons to the muscle fiber. - Troponin
Incorrect. Troponin plays a role in actin-myosin interaction, but it does not generate force. - Actin
Incorrect. Actin is the protein being pulled but does not use ATP directly. - Myosin
Correct. Myosin needs ATP to release from the actin filament and move the lever arm for a new power stroke.
What roles do calcium ions and ATP play in muscle contraction?
Our Answer: Calcium ions must be present for the myosin binding site on the G-actin to be exposed. This occurs because troponin changes shape when calcium binds to it, pulling the tropomyosin away from the myosin binding sites on actin. ATP is required in order to: 1) break cross-bridges after a cross-bridge cycle and 2. activate the myosin heads (through ATP hydrolysis).
How can medical examiners determine the time of death based on the state of rigor mortis?
Our Answer: Full rigor sets in after ATP is used up and lasts a few hours to days. After that time, proteins degrade, and rigor-induced stiffness is lost. The amount of time a body is in pre-rigor, rigor, and post-rigor depends on body type and environmental conditions.
Learn By Doing 11.8
Athletes will train differently depending on what type of sport they are engaging in. If an athlete was training to become a bodybuilder what would their main source of energy for respiration be?
- Glucose
Correct. Bodybuilding requires a lot of energy in a short amount of time. Glucose can quickly be turned into ATP using anaerobic fermentation. - Fatty acids
Incorrect. Fatty acids can enter aerobic respiration, which takes longer and requires more oxygen than is available during the short burst of exercise. - Phosphate
Incorrect. Inorganic phosphate by itself does not provide energy for muscle movement. - Protein
Incorrect. Protein can be used as a fuel during aerobic respiration, which takes longer and requires more oxygen than is available during the short burst of exercise.
Learn By Doing 11.9
Which protein is found in the thick filaments?
- Troponin
Incorrect. Troponin is part of the thin filament and binds calcium ions. - Actin
Incorrect. Actin is the central component of thin filaments. - Tropomyosin
Incorrect. Tropomyosin binds to actin in the thin filaments. - Myosin
Correct. Bundled myosin groups form thick filaments.
Which of the following describes the orientation of both thick and thin filaments in the sarcomere?
- perpendicular
Incorrect. This would mean the filaments are oriented at right angles to one another, which is not the case. - parallel
Correct. The filaments all go in the same direction with some overlap. - thin and thick filaments are found in different parts of the sarcomere
Incorrect. The filaments are found in the same parts of the sarcomere. - skewed
Incorrect. If the filaments were skewed, they could not interact properly.
Learn By Doing 11.10
Which of the following shortens during muscle contraction?
- the thin filament
- the sarcomere
- the thick filament
- actin
- myosin
Describe how the actin and myosin, and the thick and thin filaments, are related to the M line and Z line.
Our Answer: The thick filament is made of individual myosin molecules that are bound to the M line in the middle of the sarcomere. The thin filament is made mainly of actin molecules, and these are bound to the Z lines on the two edges of the sarcomere. Because of this, when the thick filaments pull the thin filaments toward the center of the sarcomere, the Z lines are drug along. This results in the shortening of the sarcomere without a change in the length of the thick or thin filament.
Did I Get This? 11.2
Below are descriptions that relate to one of the major structures of a skeletal muscle fiber. Match one of the phrases below to the proper part of the muscle fiber. Names may be used more than once. Choose the most specific term.
Muscle fiber
Myofibrils
Sarcoplasm
Sarcoplasmic reticulum
Sarcolemma
Sarcomere
Triad
Transverse tubule
- It contains two Z discs Sarcomere
- The plasma membrane of a fused skeletal muscle cell Sarcolemma
- Contains a T tubule and two terminal cisternae Triad
- Contains an M line Sarcomere
- Stores calcium ions (Ca2+) Sarcoplasmic reticulum
- An extension of the sarcolemma Transverse Tubule
- Location of myoglobin Sarcoplasm
- Site of nerve interaction Sarcolemma
- The cytoplasm of a fused skeletal muscle cell Sarcoplasm
- Similar to smooth endoplasmic reticulum Sarcoplasmic reticulum
- Composed of many thick and thin filaments Myofibril
Which of the following is correct about Ca2+ in skeletal muscle?
- It is stored in the T tubules.
- It is unique to muscle cells.
- It conducts the action potential in the sarcolemma
- It binds to troponin of the sarcomeres.
Why are the T tubules important for coordinating muscle contraction?
- They produce ATP for energy.
- They prevent the release of Ca2+ ions.
- They conduct action potentials to the interior of the cell.
- They store oxygen in hemoglobin.
Learn By Doing 11.11
Why are mature, contracting skeletal muscle cells amitotic (they do not have the ability to divide to produce new muscle cells)?
- Muscle cells have no DNA.
Incorrect. Nuclei of muscle cells (which contain DNA) are not lost, but they do move to the edges. - Muscle cells have no organelles.
Incorrect. Organelle numbers change, but muscles need lots of mitochondria for energy generation. - The cellular structures required for division get in the way of the organized sarcomeres.
Correct. Cells also require huge amounts of energy to divide; energy can be robbed by division machinery. - Muscle cells take a very long time to form, and the cells have gotten old and stopped dividing by the time they generate force.
Incorrect. The age of the cells is not a factor; their differentiation state is a factor.
During development, what cells fuse to form muscle fibers?
- Myoblasts
Correct. Myoblasts do migrate and fuse to form fibers. - Osteocytes
Incorrect. Osteocytes are mature bone cells. - Myocytes
Incorrect. Myocytes are mature muscle cells. - Satellite cells
Incorrect. Satellite cells fuse to the muscle cell.
Which of the following is not true about satellite cells?
- They are not able to divide.
Correct. Satellite cells are able to divide. - They can limitedly regenerate muscle fibers.
No, this statement is correct. They can induce limited regeneration in muscle fibers. - They can fuse with muscle cells.
No, this statement is correct. Satellite cells can fuse with muscle cells. - They can repair damage in living cells.
No, this statement is correct. Satellite cells repair damage in living cells.
Did I Get This? 11.3
Which of the following is a neurotransmitter?
- troponin
No, troponin is a protein located in the muscle fiber. - acetylcholine
Yes, Acetylcholine is the neurotransmitter involved at the neuromuscular junction. - sodium ions
No, sodium ions move into the muscle fiber after acetylcholine binds, causing an action potential.
Which of the following is the correct order of a signal being passed from a neuron to a muscle?
- Motor end plate, synaptic cleft, axon terminal
Incorrect. The motor end plate is part of the muscle fiber. - Synaptic cleft, motor end plate, axon terminal
Incorrect. The synaptic cleft is in between the presynaptic membrane and the motor end plate. - Axon terminal, synaptic cleft, motor end plate
Correct. The axon terminal is presynaptic, then the synaptic cleft, and then the motor end plate of the muscle fiber. - None of these is in the correct order.
Incorrect. One of these choices is the correct order of signaling.
Learn By Doing 11.12
What would happen to a person who had abnormally high Ca2+ concentration in their sarcoplasm?
- They would not be able to contract their muscles.
Incorrect. With excess Ca2+ there would be unwanted muscle contractions. - The action potential would not be propagated.
Incorrect. Na+ ions are involved in propagating the action potential, not calcium. - The troponin would not let the myosin bind.
Incorrect.Ca2+ will allow the myosin to bind with actin. - They would have unwanted muscle contractions.
Correct. Too much Ca2+ will allow the myosin to bind the actin at unwanted times resulting in unwanted muscle contraction.
Learn By Doing 11.13
Stimulation of additional motor units will increase the strength of contraction. This process is called:
- tetanus
- summation
- contraction
- recruitment
Explain the difference between a muscle twitch and a muscle spasm.
OUR ANSWER: A muscle spasm is a result of an entire muscle or muscle group contracting involuntarily. A muscle twitch is produced by the stimulation of all muscle fibers in a motor unit and does not produce a significant amount of muscle contraction. You would need multiple involuntary “muscle twitches” to create a muscle spasm.
Learn By Doing 11.14
Negative feedback occurs when environmental conditions move the body away from optimal conditions. For example, as your body cools below 98.6°F (37°C), the control center in your brain signals that actions need to be taken to raise your body temperature. The muscular system will begin to act. The smooth muscle of the blood vessels in the extremities will constrict and shut the blood to the important organs. What phenomena does this explain:
- One reason that frostbite occurs on the fingers and nose first.
Correct. Your fingers, toes, and nose are most likely to experience frostbite from cold first. - The feeling of hyperthermia in your extremities.
Incorrect. Hyperthermia is the result of too much heat. The warm blood would be moved to your important organs and away from your extremities. - The occurrence of varicose veins in the legs.
Incorrect. Varicose veins are the result of faulty valves in the legs. - The excessive thirst you feel when you are hot.
Incorrect. Your thirst does not have anything to do with the blood being shunted to your important organs.
Why will people die if their body temperature increases too much?
- The body will undergo spontaneous combustion.
- The body’s metabolic reactions are interrupted.
- The body’s metabolic reactions will begin to go too fast.
- The body will not be able to get enough calcium.
In older people, the ratio of muscle fibers to connective tissue decreases. This may result in
- Less powerful movements
- More powerful movements
- More motor units being activated
- An increase in muscle tension
Endurance training can cause angiogenesis which is an example of how structure and function are related because
- There is an increase in adipose tissue that increases the endurance of the muscle.
- There is an increase in capillaries that decreases the endurance of the muscles.
- There is an increase in muscle fibers that increases the atrophy of the muscle.
- There is an increase in capillaries that increases the endurance of the muscles.
Learn By Doing 11.15
Which of the following depends on the proper function of skeletal muscles?
- Walking
Partially correct. This is one of the actions that depend on the proper functioning of skeletal muscle. - Talking
Partially correct. This is one of the actions that depend on the proper functioning of skeletal muscle. - Blood flow
Partially correct. This is one of the actions that depend on the proper functioning of skeletal muscle. - All of the above
Correct; all of the above rely on the skeletal muscle functioning properly.
Varicose veins occur when valves are not functioning properly and blood that should be flowing toward the heart pools in the vein. Varicose veins are more common in the legs. Which of the following is the best explanation for this?
- Varicose veins are more common in the legs because there are larger veins.
Incorrect. The largest veins are located in the stomach, chest, and neck. - Varicose veins are more common in the legs because there is more blood in the legs.
Incorrect. The volume of blood does not cause the varicose veins - Varicose veins are more common in the legs
Incorrect. The legs are further from the heart and the blood must flow against gravity to return to the heart. - Varicose veins are more common in the legs because there are more valves in the legs.
Correct. Because the blood must flow against gravity to return to the heart, there are more valves in the legs to prevent backflow.
Which of the following is not matched with its proper function in the cardiovascular system:
- Cardiac muscle- contractions of the heart pump blood to the body.
Incorrect. The cardiac muscle of the heart pumps blood to the rest of the body. - Smooth muscle- contraction and relaxation allow the shunting of blood to where it is needed.
Incorrect. The smooth muscle of the blood vessels is responsible for shunting blood - Skeletal muscle- contractions around major arteries help move blood to the body.
Correct. Skeletal muscle assists the major veins in moving the blood back to the heart
Which of the following is accomplished using smooth muscle?
- Chewing food
Incorrect. This involves voluntary skeletal muscles. - Mixing food
Correct. This involves involuntary smooth muscles. - Biting food
Incorrect. This involves voluntary skeletal muscles. - Voluntary control of the anal sphincter
Incorrect. This involves voluntary skeletal muscles.