Levels of Organization
6 Biology of the Cell
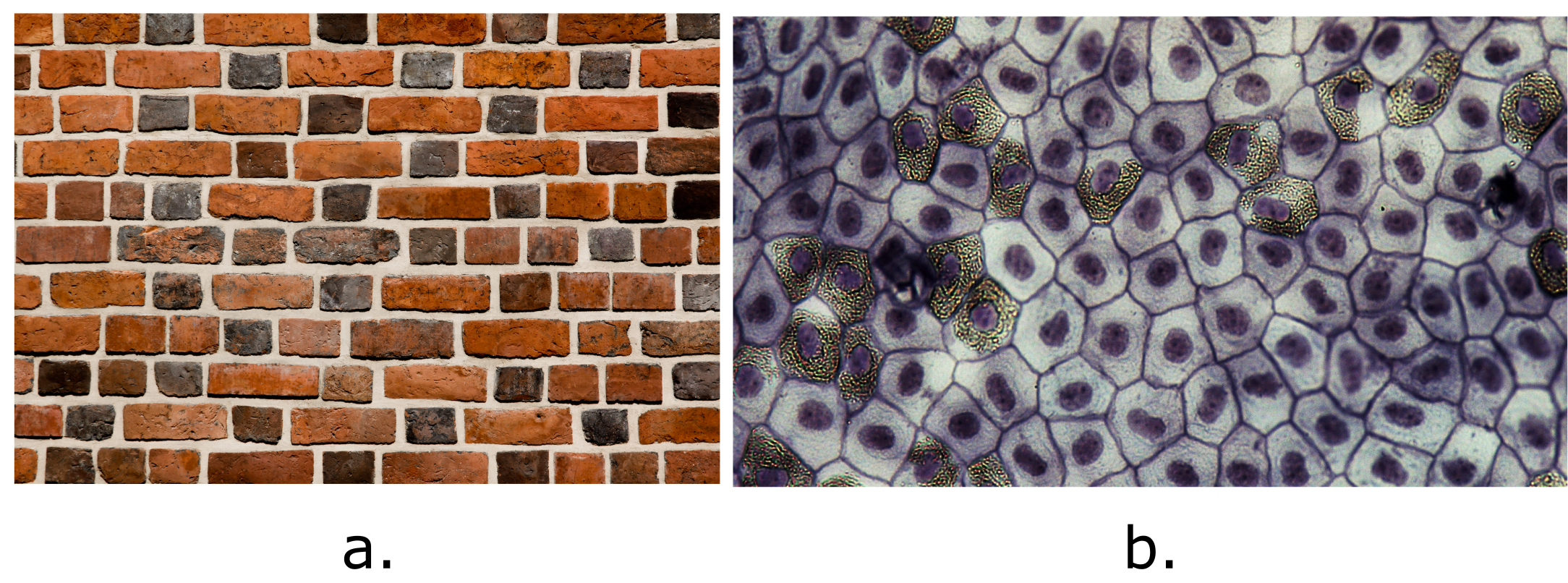
Close your eyes and picture a brick wall. What is the basic building block of that wall? It is a single brick, of course. Like a brick wall, your body is composed of basic building blocks, and the building blocks of your body are cells. Your body has many kinds of cells, each specialized for a specific purpose. Just as a home is made from various building materials, the human body is constructed from many cell types. For example, epithelial cells protect the body’s surface and cover the organs and body cavities. Bone cells help to support and protect the body. Cells of the immune system fight invading bacteria. Red blood cells carry oxygen throughout the body. Each cell type plays a vital role in our body’s growth, development, and day-to-day maintenance. Despite their enormous variety, however, all cells share certain fundamental characteristics.
Cell Theory
Learning Objectives
- Define the term cell.
- Summarize the three principle points of the Cell Theory
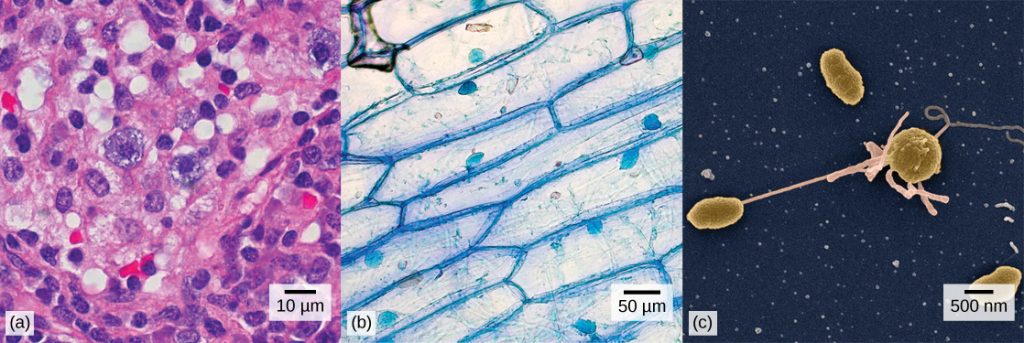
Cells are the basis of life—the basic structural unit of living things. Molecules such as water and amino acids are not alive, but cells are. And all life is comprised of cells of one type or another. One of the hallmarks of living systems is the ability to maintain homeostasis, or a relatively constant internal state. For example, living cells require a water-based environment to survive; various physical (anatomical) and physiological mechanisms keep the human body’s trillions of cells immersed in fluid. This is one aspect of homeostasis. When a particular parameter, such as blood pressure or oxygen content, moves far enough out of homeostasis (generally becoming too high or too low), illness or disease—and sometimes death—inevitably results. The cell is the first level of complexity able to maintain homeostasis, and it is the unique structure of the cell that enables this critical function. In this section of the course, you will learn about the cell and all the parts that make it functional.
The concept of a cell started with microscopic observations of dead cork tissue by scientist Robert Hooke in 1665. Without realizing their function or importance, Hook coined the term “cell” based on the resemblance of the small subdivisions in the cork to the rooms that monks inhabited, called cells. About ten years later, Antonie van Leeuwenhoek became the first to observe living and moving cells under a microscope. In the century that followed, the theory that cells represented the basic unit of life would develop. These tiny fluid-filled sacs house components responsible for the thousands of biochemical reactions necessary for an organism to grow and survive. The Cell Theory guides our modern understanding of cells. It has three parts:
- All known living things are composed of one or more cells.
- All new cells are created by pre-existing cells dividing in two.
- The cell is the most basic unit of structure and function in all living organisms.
Modern cell theorists assert that all functions essential to life occur within the cell and that, during cell division, the cell contains and transmits to the next generation of cells the information necessary to conduct and regulate cell functioning.
Learn By Doing 6.1
True or false: Cells are alive.
Hint: Living structures maintain homeostasis and replicate.
The human body contains bacterial cells and human cells. In fact, bacterial cells outnumber human cells 10 to one. Both are considered cells because they:
Hint: Think about which choice fits into cell theory.
- have membranes
- can divide into new cells
- are made of biomolecules
What does the cell theory propose?
- All organisms are made of one or more cells.
- All the life functions of an organism occur within cells.
- All cells come from existing cells.
- All of the above
Comparing Prokaryotic and Eukaryotic Cells
Learning Objectives
- Identify and briefly describe the functions of the four main parts of all cells.
- Name examples of prokaryotic and eukaryotic organisms
- Compare and contrast prokaryotic cells and eukaryotic cells
- Describe the relative sizes of different kinds of cells
Even though all cells share certain characteristics, there is incredible diversity in structure and function among different cells. The human body alone contains trillions of cells of more than 200 different types, each with a unique structure and function. Yet cell structure can be categorized into two broad types, prokaryotes and eukaryotes, that share some characteristics but are very different in others. These similarities arise from the common descent of all life from a single ancestor approximately 3.8 billion years ago (bya). The differences arise from different evolutionary pathways taken by life after its beginning. We refer to an organism’s history as its phylogeny.
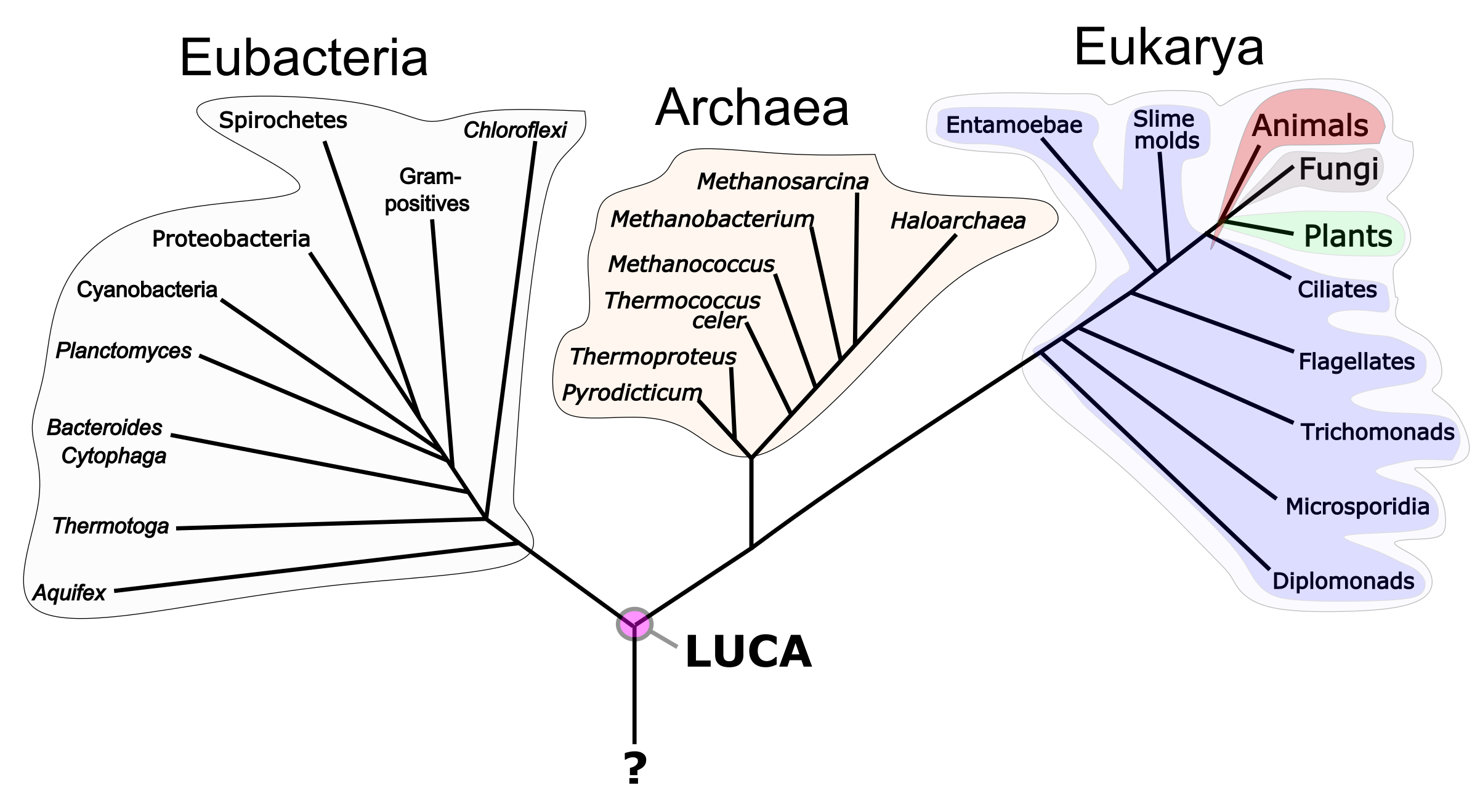
Our current “tree of life” contains three major branches called domains (Figure 6.3). These are the Eubacteria (eu- = true), Archaea, and Eukarya (-karyon- = kernel). Three branches contain within them the entire variety of microscopic and macroscopic life. This way of showing evolutionary history is a tool called a phylogenetic tree. It shows evolutionary pathways and connections among organisms. The bottom of the tree represents the beginning of life, an event that is the subject of much research. As of yet, we have no answer to the question of how life arose from non-life. Moving up the tree trunk, we find an organism referred to as LUCA— the last universal common ancestor of all living organisms. The location where a split occurs is called a branch point and represents where a single lineage evolved into a distinctly new one. The tips of the branches represent currently living organisms. Phylogenetic trees can serve as a pathway to understanding evolutionary history. The pathway can be traced from the origin of life to any individual species by navigating the evolutionary branches between the two points. Also, by starting with a single species and tracing back towards the “trunk” of the tree, one can discover that species’ ancestors and where lineages share a common ancestry.
The Eubacteria and Archaea are prokaryotes, structurally simple, single-celled organisms lacking intracellular organelles. Our species homo, sapiens, is located within Eukarya. This domain is split into four kingdoms: animals, plants, fungi, and protists. Amoebae (singular, amoeba) are an example of a protist. The small branch that plants and animals (including humans) occupy in this diagram shows how recent and minuscule these groups are compared with other organisms. Eukaryotic cells are larger and more internally complex than the prokaryotic cells of Eubacteria and Archaea. Before discussing the differences between eukaryotic and prokaryotic cell structures, we will first discuss their commonalities.
All cells must possess several characteristics to maintain homeostasis. We might think of this as the basic “toolkit” required for life. There must be a way of separating the cell’s chemistry from that surrounding the cell. There must be a medium conducive to the chemistry of life. There must be a source of information on how to replace or repair worn-out molecules. There must be molecular machines capable of using that information. Finally, there must be a source of energy to power the anabolic chemistry of life. Not surprisingly, all cells share four common components: 1) a plasma membrane, which is an outer covering that separates the cell’s interior from the surrounding environment; 2) cytoplasm, which consists of a jelly-like aqueous region inside the cell; 3) DNA, which is the genetic information for building the molecular machines necessary for life; and 4) ribosomes, small molecular machines that synthesize proteins. However, there are several important differences between prokaryotic and eukaryotic cells. One of these differences lies in how different types of cells capture energy from their environment. We’ll discuss that in more detail later in this chapter. First, we look at the more noticeable difference between the two types of cell structure.
Components of Prokaryotic Cells
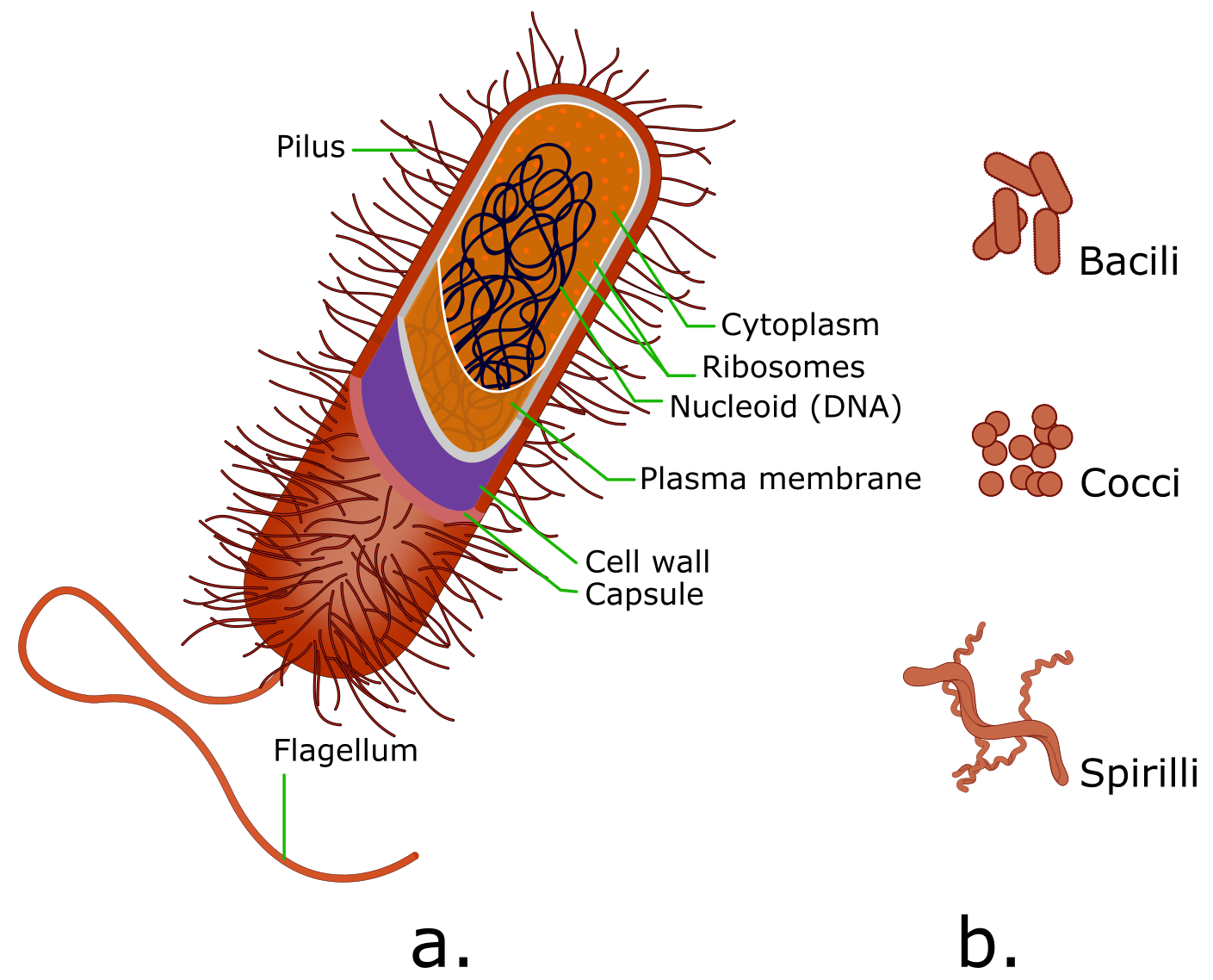
The unicellular organisms of the domains Eubacteria and Archaea are classified as prokaryotes (pro- = before; -karyon- = nut). A prokaryotic cell is a structurally simple, single-celled organism without any membrane-bound organelles. Prokaryotic cells have a circular chromosome concentrated in a region called the nucleoid (Image 6.4a). Prokaryotic cells occur in a limited number of shapes, rod-like bacilli, spherical cocci, and twisted spirilli (Image 6.4b). Eukaryotes are significantly different in all three respects.
Unlike Archaea and some of the Eukarya, bacteria have a cell wall made of sugars and amino acids, and many have a polysaccharide capsule. These act as an extra layer of protection, helps the cell maintain its shape, and prevents dehydration. The capsule enables the cell to attach to surfaces in its environment. Some prokaryotes have flagella or pili. Flagella are used for locomotion, while most pili are used to exchange genetic material during a type of reproduction called conjugation.
Components of Eukaryotic Cells
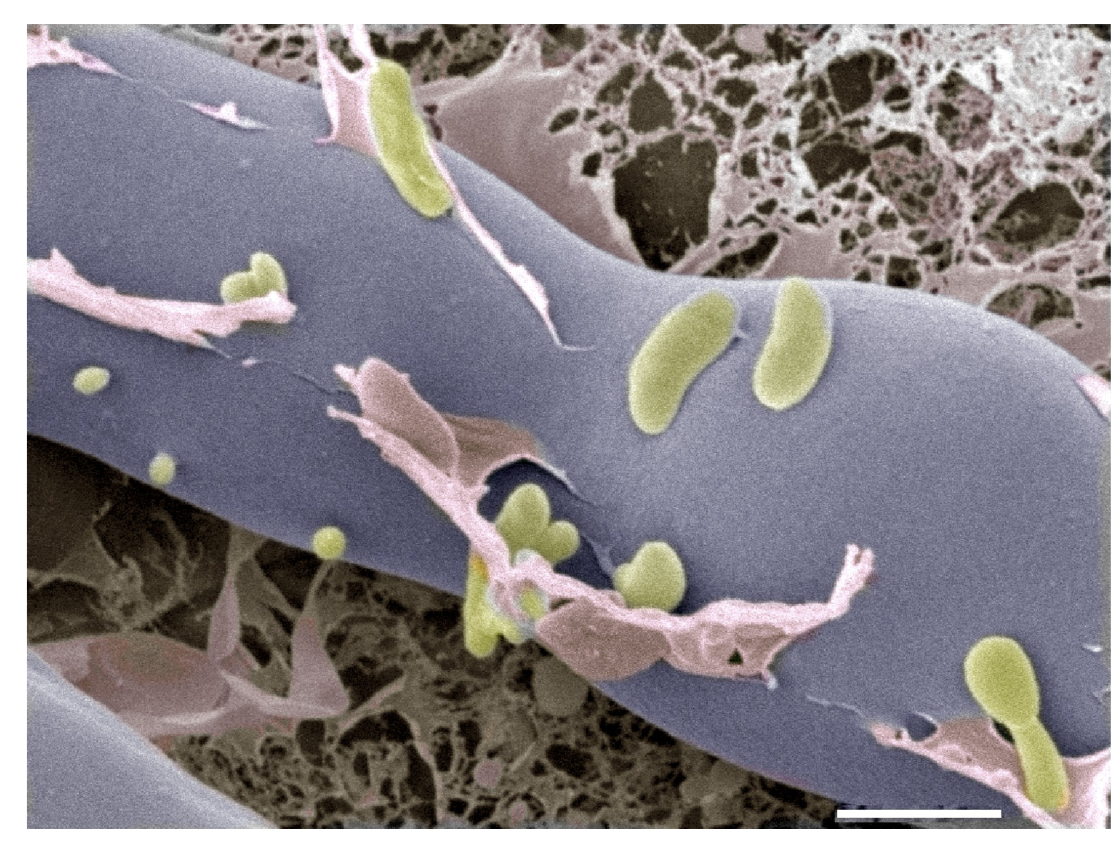
Eukaryotic cells are about 10 to 15 times wider than the typical prokaryotic cell and up to 1,000 times greater in volume. As mentioned earlier, there are four kingdoms within the domain Eukarya– animals, plants, fungi, and protists. As different as organisms in the four kingdoms seem, eukaryotic cells are large with complex external and internal architecture. The protists are unicellular rather than multicellular but share the same set of membrane-bound compartments or sacs called organelles, which have specialized functions. An example of a protist that you might be familiar with is the amoebae The word eukaryotic means “true kernel” or “true nut,” alluding to the presence of the membrane-bound nucleus in these cells. The term “organelle” means “little organ.” Organelles have specialized cellular functions, just as the organs of your body have specialized functions. We will review eukaryotic organelles later in the chapter.
Section Summary
Prokaryotes are predominantly single-celled organisms of the domains Eubacteria and Archaea. All prokaryotes have plasma membranes, cytoplasm, ribosomes, and DNA but lack membrane-bound organelles. Many also have polysaccharide capsules. Prokaryotic cells range in diameter from 0.1–5.0 µm.
Like prokaryotes, the cells of Eukaryotic organisms have a plasma membrane, DNA, cytoplasm, and ribosomes, but a eukaryotic cell is typically larger than a prokaryotic cell, has a true nucleus (meaning its DNA is surrounded by an additional phospholipid membrane), and has other membrane-bound organelles that allow for compartmentalization of functions. Eukaryotic cells tend to be 10 to 100 times the size of prokaryotic cells. Table 6.1 summarizes the differences between prokaryotic and eukaryotic cells.
Characteristic |
Prokaryotes |
Eukaryotes |
Genetic information | DNA is circular, usually free-floating in cytoplasm | DNA is linear, found in the nucleus |
Complexity | No nucleus or membrane-bound organelles | Has nucleus and membrane-bound organelles (i.e., mitochondria, chloroplasts, Golgi body, ER) |
Size | Small (1-5 micrometers) | Larger (10-100 micrometers) |
Domains | Eubacteria and Archaea | Eukarya: the four kingdoms are animals, plants, fungi, protists |
Cell Number | Always unicellular | May be unicellular or multicellular |
Learn By Doing 6.2
Which of these do all prokaryotes and eukaryotes share?
- nuclear envelope
- cell walls
- organelles
- plasma membrane
A typical prokaryotic cell __________________ compared to a eukaryotic cell.
- is smaller in size by a factor of 100
- is similar in size
- is smaller in size by a factor of one million
- is larger in size by a factor of 10
Antibiotics are medications used to fight bacterial infections in humans and other eukaryotes. Consider the following statements; which one is probably true about antibiotics?
- Antibiotics are poisonous to all cells.
- Antibiotics are only poisonous to prokaryotic cells.
- Antibiotics are only poisonous to eukaryotic cells.
You find a cell and examine it under a microscope. You can see that the cell has a nucleus. What can you conclude about this cell?
- This cell is a eukaryote.
- You cannot draw conclusions given only this information.
- This cell is a prokaryotic cell.
Which of the following structures is found in BOTH prokaryotes and eukaryotes? (More than one answer is correct.)
- cytoplasm
- ribosome
- nucleus
- DNA
- Mitochondria
Cell Size
If cells are the fundamental unit of life, why are they so small? And why, when multicellular organisms grow, do they add additional cells through cell division rather than simply increasing the size of the cells already present? The answers to these questions lie in a cell’s need for fast, easy food. The need to be able to pass nutrients and gases into and out of the cell sets a limit on how big cells can be. The larger a cell gets, the more difficult it is for nutrients and gases to move in and out of the cell.
This is because as a cell grows, the amount of material within the cell (volume) increases more quickly than the amount of membrane (total surface area) available to service the cell’s needs. If a cell was to get very large, the small surface area would not allow enough nutrients to enter the cell quickly enough for the cell’s needs.
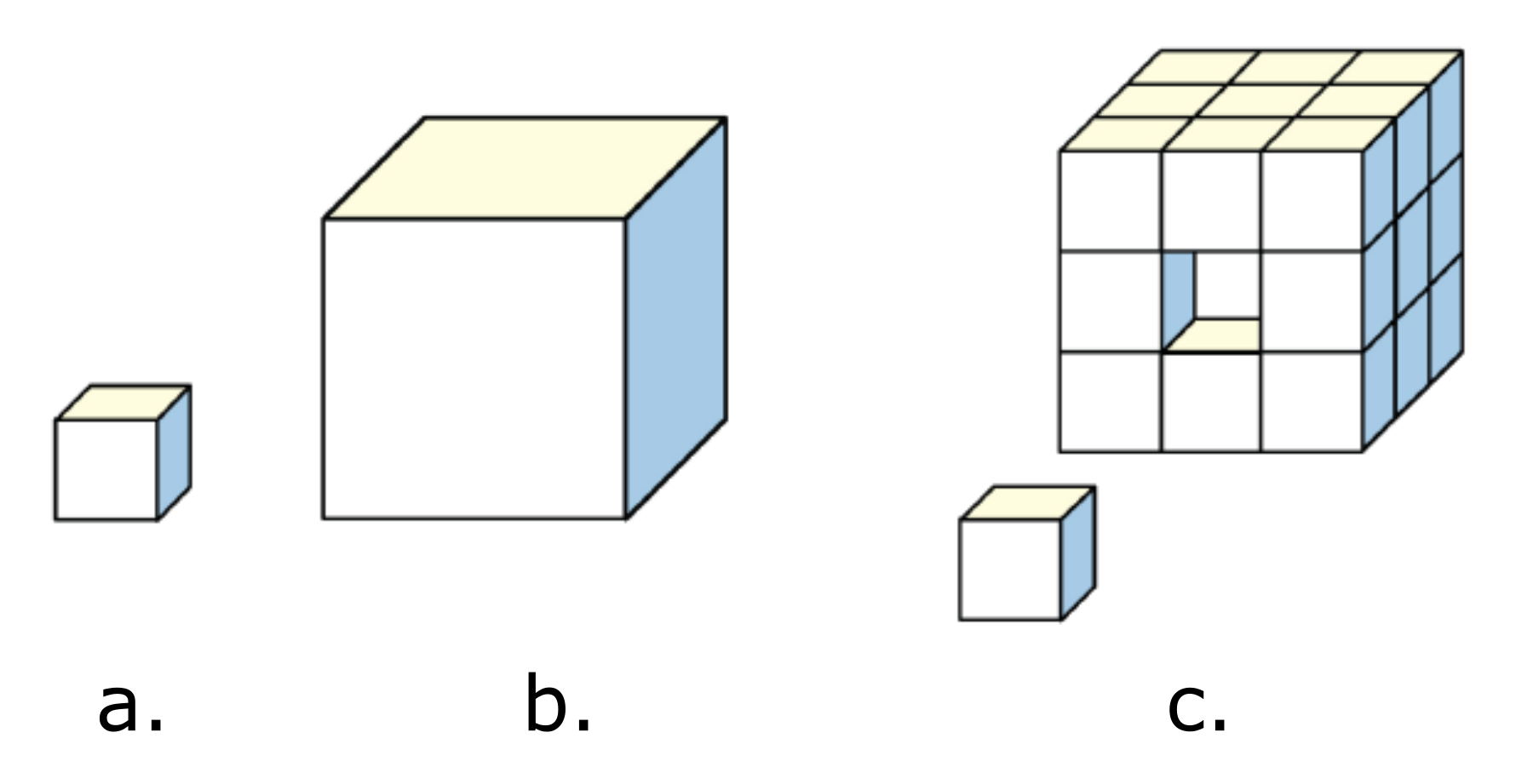
To explore the relationship between the volume of material inside a cell and the amount of cell membrane available to transport food and waste, we can imagine cells as tiny cube-like boxes. Since cells aren’t actually cubes with all sides the same size, this is an oversimplification. However, this is a useful exercise since it clearly demonstrates the relationship between surface area and volume without the need for complex math. This idea is illustrated in Figure 6.5 and described in detail below.
Let us imagine a small cube-like cell is one unit in length (Figure 6.5 a). The total surface area of this cuboidal cell represents the amount of cell membrane and is calculated by the equation: (height × width) × number of sides. In this example, the equation for the total surface area is solved as (1 unit × 1 unit) × 6 sides = 6. The volume of the cuboidal cell represents the amount of cytoplasm and other materials inside the membrane. The volume of a cube is calculated as (height x width x length). In this example, the equation is solved as (1 unit × 1 unit x 1 unit) = 1. The amount of membrane in relation to the internal materials in the cell can be described as the ratio of total surface area to volume, or surface area ÷ volume. In our cuboidal cell, this ratio is 6 ÷ 1 = 6.
Now let’s imagine a larger cell that is 3 units in length. Using the same equations, we would have a total surface area of (3 units × 3 units) × 6 sides = 54 and a volume of 3 units × 3 units × 3 units = 27. The surface area to volume ratio of the larger cell is then 54 ÷ 27 = 2.
So we can see that increasing cell size isn’t a viable way for an organism to grow as it dramatically reduces the surface area to volume ratio. But what if we replace the three-unit cell with enough one-unit cells to equal the volume of the single three-unit cell? This can be done with 27 one-unit cells. The total surface area of the 27 cells would then be [(1 unit × 1 unit) × 6 sides] × 27 cells = 162. The total volume of the block of 27 cells is given by (1 unit × 1 unit x 1 unit) × 27 cells = 27. The surface area to volume ratio of the 27 cells is 162 ÷ 27 = 6, the same ratio as our 1 unit cell! It’s important to understand that this works because all living cells are bathed in fluids that allow for gas and nutrient transport. An increased surface area to volume ratio means increased exposure to the environment. This in turn means that nutrients and gases can move in and out of a small cell more easily than in and out of a larger cell.
Larger cells have evolved ways of working around certain size challenges. Big cells, such as osteoclast bone cells and skeletal muscle fibers, are formed when multiple precursor cells fuse. This results in multiple nuclei, allowing such cells to supply enough messenger RNA and proteins for the cell’s needs. Large, metabolically active cells often have lots of folds in their cell surface membrane. These folds increase the surface area available for transport into or out of the cell. Such cell types are found lining your small intestine, where they absorb nutrients from your food through little folds called microvilli.
Section Summary
As a cell increases in size, the surface area-to-volume ratio decreases. If the cell grows too large, the plasma membrane will not have a sufficient surface area to support the rate of diffusion required for the increased volume since volume increases more rapidly than surface area.
Learn By Doing 6.3
The physical characteristics of a cell influence how it functions. For example, the surface area and volume of a cell affect how efficiently waste is removed from the cell via diffusion.
The table below shows data for four different cuboidal cells.
Cell | Length of One Side () |
A | 1 |
B | 2 |
C | 3 |
D | 4 |
Using the information from the table, what is the surface-area-to-volume ratio for the cuboidal cell that removes waste most efficiently?
Hint: The length, width, and height of a cube are all the same.
Calculate the surface area, volume, and surface area to volume ratio for each cell and then compare them.
Did I Get This? 6.1
The geometric explanation for why cells are small is that as cell size increases, the
- volume and surface area decrease.
- volume increases faster than the surface area.
- surface area and volume increase at the same rate.
- surface area increases faster than the volume.
Unique Features of Eukaryotic Cells
The life of a bee is very different from the life of a flower, but the two organisms are related. Both are members of the domain Eukarya and have cells containing many similar organelles, genes, and proteins. Animals and plants are familiar eukaryotes; in fact, all large complex organisms are eukaryotes. Fungi (the singular form is “fungus“), which include yeasts, molds, and mushrooms, are also eukaryotes. There are even single-celled eukaryotes called protists. These four groups, animals, plants, fungi, and protists, make up the four kingdoms within the domain Eukarya.
Let’s continue our study of the cell by investigating the basic anatomy of a eukaryotic cell. Each cell consists of three general components, indicated to some extent in Figure 6.7.
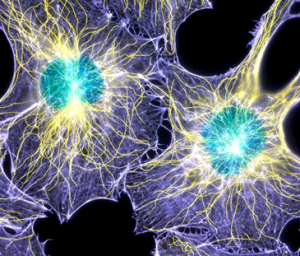
-
- A cell membrane (also called a plasma membrane) surrounds and protects the cell
- The cytoplasm, which is the watery interior of the cell and contains ions, proteins, and organelles
- Organelles carry out all activities necessary for the cell to live, grow, and reproduce.
Within the body, cells represent a level of organization between organelles and tissues. Organelles, in turn, are comprised of specialized macromolecules, whereas tissues are collections of specialized cells. Tissues can vary between organs and within organs based on the structure and function of the cells of which they are made. Thus, each tissue type’s cells vary in shape, size, and interior structure to permit their specific physiological function within the tissue. As you study Human Biology, one important concept to remember is that structure determines function. When you look at the shape of a cell, it gives you a clue to its function.
Cytoplasm
The cytoplasm is the entire region between the plasma membrane and the nuclear envelope (a structure we will discuss shortly). It is comprised of organelles suspended in the gel-like cytosol, the cytoskeleton, and various chemicals. Even though the cytoplasm consists of 70 to 80 percent water, it has a gel-like consistency, which comes from the proteins within it. However, proteins are not the only organic molecules in the cytoplasm. Glucose and other simple sugars, polysaccharides, amino acids, nucleic acids, fatty acids, and glycerol derivatives are also present. Ions of sodium, potassium, calcium and many other elements also dissolve in the cytoplasm. Many metabolic reactions, including protein synthesis, take place in the cytoplasm. The most important function of the cytosol, then, is metabolism.
Plasma Membrane
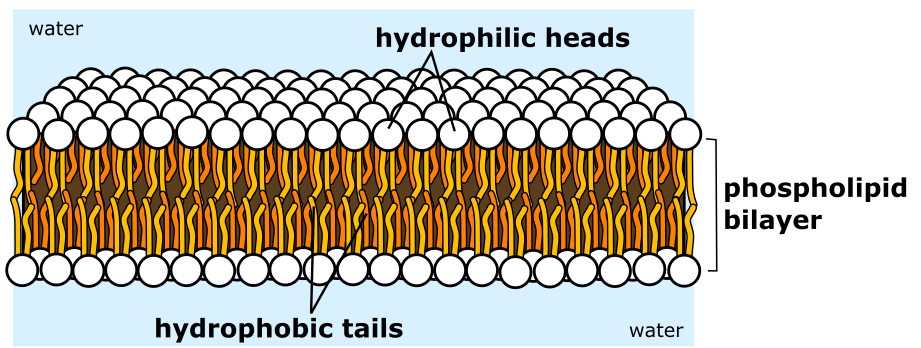
The plasma membrane structure is a mosaic of components—including phospholipids, cholesterol, proteins, and carbohydrates—that gives the membrane a fluid character. Plasma membranes range from 5 to 10 nm in thickness. For comparison, human red blood cells, visible via light microscopy, are approximately 8 µm wide, or about 1,000 times wider than a plasma membrane. Because of its mosaic structure, the plasma membrane is selectively permeable. This means that the membrane allows some materials to enter or leave the cell freely. In contrast, other materials cannot move freely but require a specialized structure and occasionally even energy investment to cross. The phospholipid bilayer is the main fabric of the membrane. The bilayer’s structure causes the membrane to be semi-permeable. We will explore the plasma membrane in more detail in the next chapter.
Organelles
Learning Objective
What would it be like to live in a one-room cabin? Well, things would probably be pretty simple. You would eat, sleep, work, and relax in a single room—which might be a bit cramped, but it would certainly make cleaning the house a snap! Prokaryotic cells, the simple cells of organisms like bacteria, are sometimes compared to one-room cabins: they don’t have internal membranes, so they’re like a single room with no walls to carve it up. Suppose we extend this analogy to eukaryotic cells, the more complex cells that make-up plants, protists, fungi, and animals. In that case, we’ll find they’re a definite step upward in the real estate market. Just as a large family home is split into many rooms with different purposes (bedrooms, bathrooms, kitchen, living room, etc.), eukaryotic cells contain various compartments with specialized functions, neatly separated from one another by layers of membrane. This organization lets each compartment maintain its own conditions, the ones it needs to carry out its job. These tasks include energy production, protein synthesis, reproduction, and processing nutrients and waste. The various cell types differ in organelle arrangement and structure. Additionally, the number of organelles of each type in a given cell varies depending on the function of the cell.
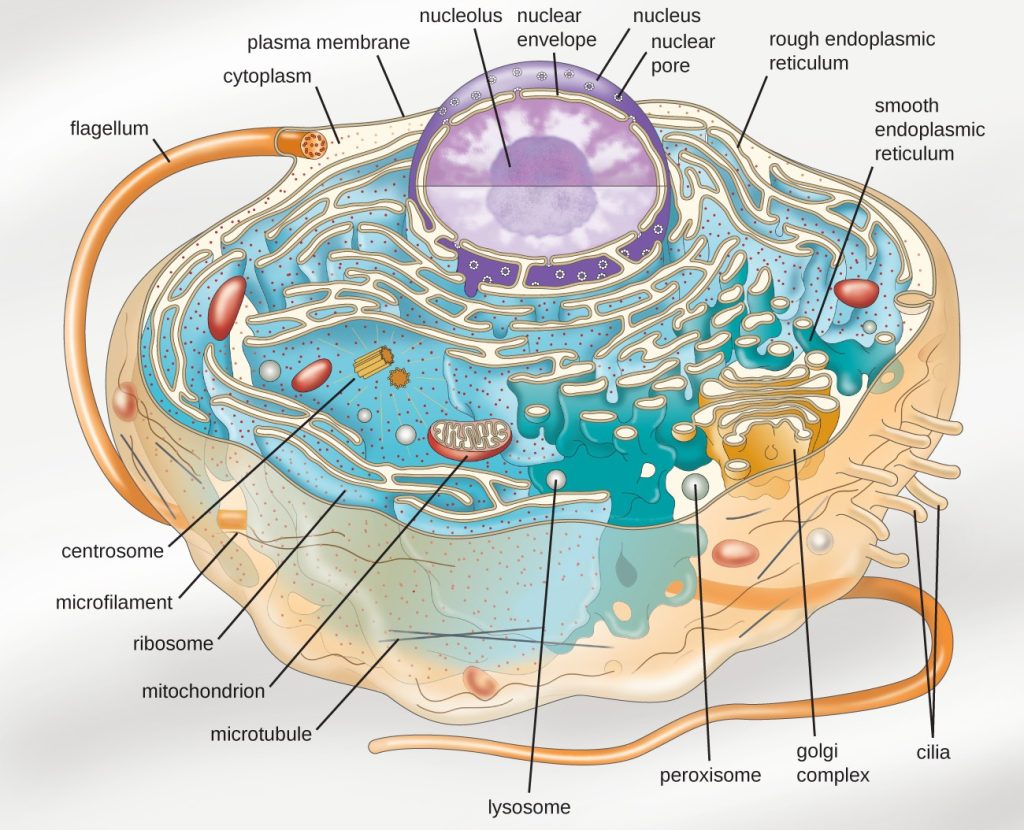
The focus of this section is to explain where organelles are commonly found within a cell, how they interact with each other, and how they function during transport, growth, and division in the cell. You will learn about the controlled chemical environment a cell maintains and what restrictions this places on the types of chemical reactions it can perform. This background is vital to understanding key processes, such as how cells release energy from glucose, make and fold proteins, and go through growth and cell division. Each process is carried out in a specific location in the cell, with most processes occurring in or around an organelle. Think of an organelle as a level of organization between macromolecules and the cell. Organelles carry out specialized tasks within the cell, localizing functions such as replication, energy production, protein synthesis, and food and waste processing. We can imagine the cell as a factory that requires certain types of structures to carry out specialized functions. A cell is similar, with each organelle serving a specific purpose.
Nucleus, Nucleolus, and Chromatin
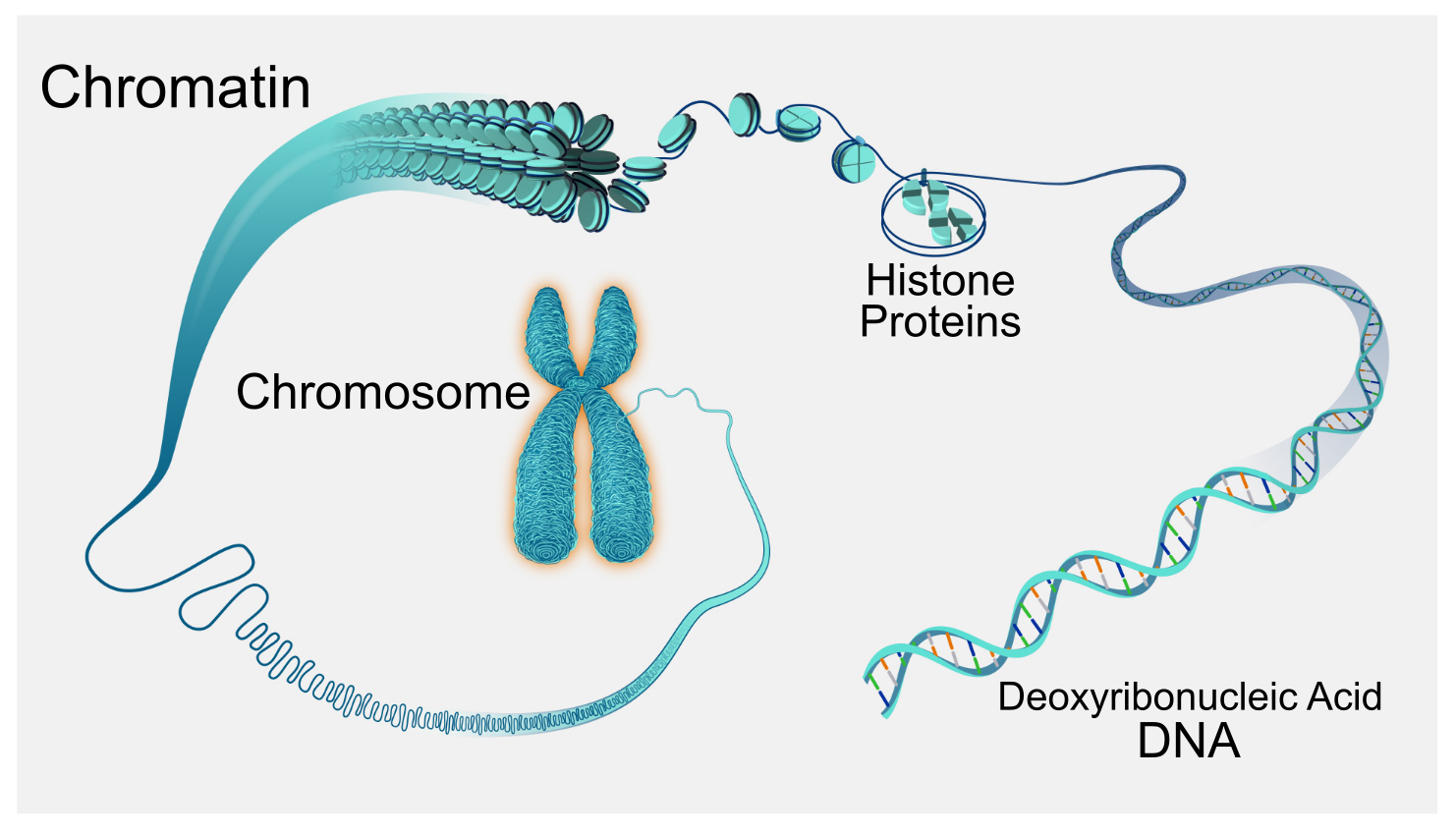
The nucleus is the control center of the cell and is usually the most prominent organelle. The nucleus (plural = nuclei) houses the cell’s genetic material. This DNA contains the instructions for how to build all the protein products required by the cell. To understand chromatin, it is helpful to first explore chromosomes. You may remember that in prokaryotes, DNA is organized into a single circular chromosome. In eukaryotes, chromosomes are linear structures. Every eukaryotic species has a specific number of chromosomes in the nucleus of each cell. For example, in humans, the chromosome number is 46, while in fruit flies, it is eight. Chromosomes are only visible and distinguishable from one another when the cell is getting ready to divide. When the cell is in the growth and maintenance phases of its life cycle, the genetic material is organized into chromatin. Chromatin is composed of DNA wound around proteins called histones (Figure 6.10). Although all cells within an individual contain the same set of chromosomes, not all areas of DNA are used to build proteins in every cell. DNA that is not required in a particular cell type is organized into heterochromatin (green), the more condensed form of chromatin. DNA used to build proteins in a cell is less condensed, a form called euchromatin. Euchromatin resembles an unwound, jumbled bunch of threads.
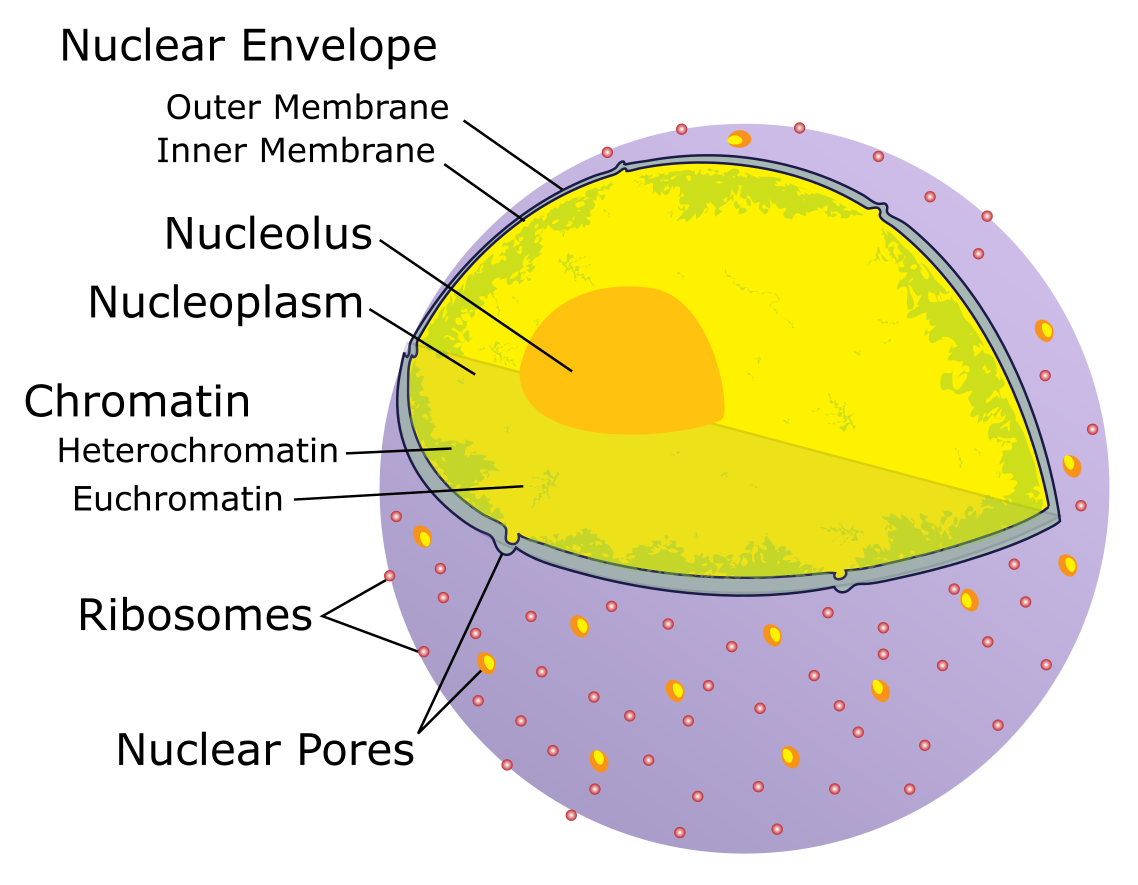
Let’s look at the nucleus in more detail in Figure 6.11. The chromatin is embedded in a gel-like substance called the nucleoplasm. The most visible structure in the nucleus is the nucleolus. In contrast to other organelles, the nucleolus is not bound by a membrane. Instead, it is an aggregate of molecules where ribosomes, another type of non-membranous organelle, are assembled. The nucleus directs the synthesis of ribosomes, but how does it do this? Some chromosomes have sections of DNA that encode ribosomal RNA. A darkly staining area within the nucleus called the nucleolus (plural = nucleoli) aggregates the ribosomal RNA with associated proteins to assemble the ribosomal subunits that are then transported out through the pores in the nuclear envelope to the cytoplasm. The nucleolus is a condensed chromatin region where ribosome synthesis occurs.
We call the nucleus’ boundary the nuclear envelope. It consists of two phospholipid bilayers: an outer and an inner membrane. The nuclear membrane is continuous with the endoplasmic reticulum (see below). Nuclear pores allow substances to enter and exit the nucleus. You can imagine that a factory command center would not be very effective if it did not have doors or windows through which to pass information to the rest of the factory.
Ribosomes
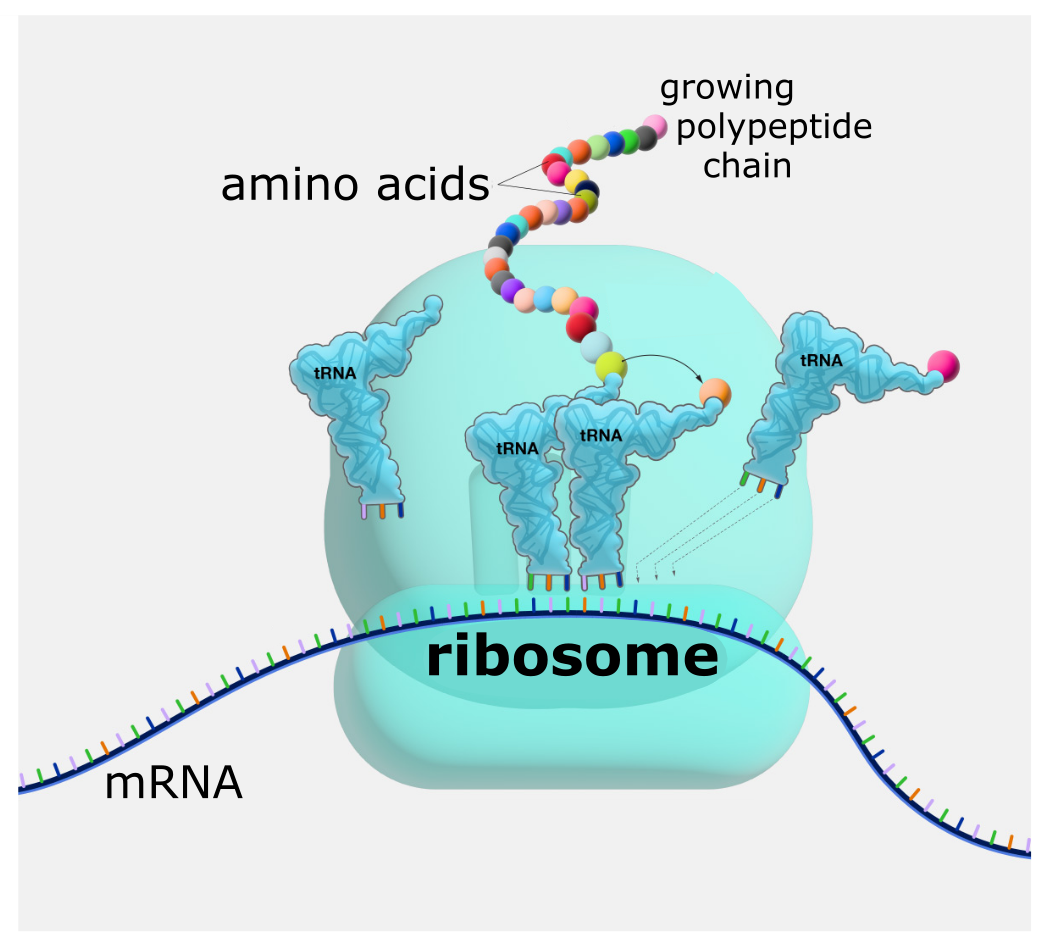
Ribosomes are amazing nanoscopic structures that are responsible for taking a genetic “blueprint” and using it to build a protein. (Nanoscopic means one thousand times smaller than microscopic!) When we view them through an electron microscope, ribosomes appear either as clusters (polyribosomes) or single, tiny dots that float freely in the cytoplasm. They can also be attached to the plasma membrane’s cytoplasmic side, the endoplasmic reticulum’s cytoplasmic side, and the nuclear envelope’s outer membrane. Ribosomes, which are large protein and RNA complexes, consist of two subunits, large and small. Ribosomes receive their “orders” for protein synthesis from the nucleus, where the DNA transcribes into messenger RNA (mRNA). The mRNA travels to the ribosomes, which translate the code provided by the sequence of the nitrogenous bases in the mRNA into a specific order of amino acids in a protein. As you know, amino acids are the building blocks of proteins.
Because protein synthesis is an essential function of all cells (including enzymes, hormones, antibodies, pigments, structural components, and surface receptors), there are ribosomes in practically every cell. Ribosomes are particularly abundant in cells that synthesize large amounts of protein. For example, the pancreas is responsible for creating several digestive enzymes, and the cells that produce these enzymes contain many ribosomes. Thus, we see another example of form following function.
Endomembrane System
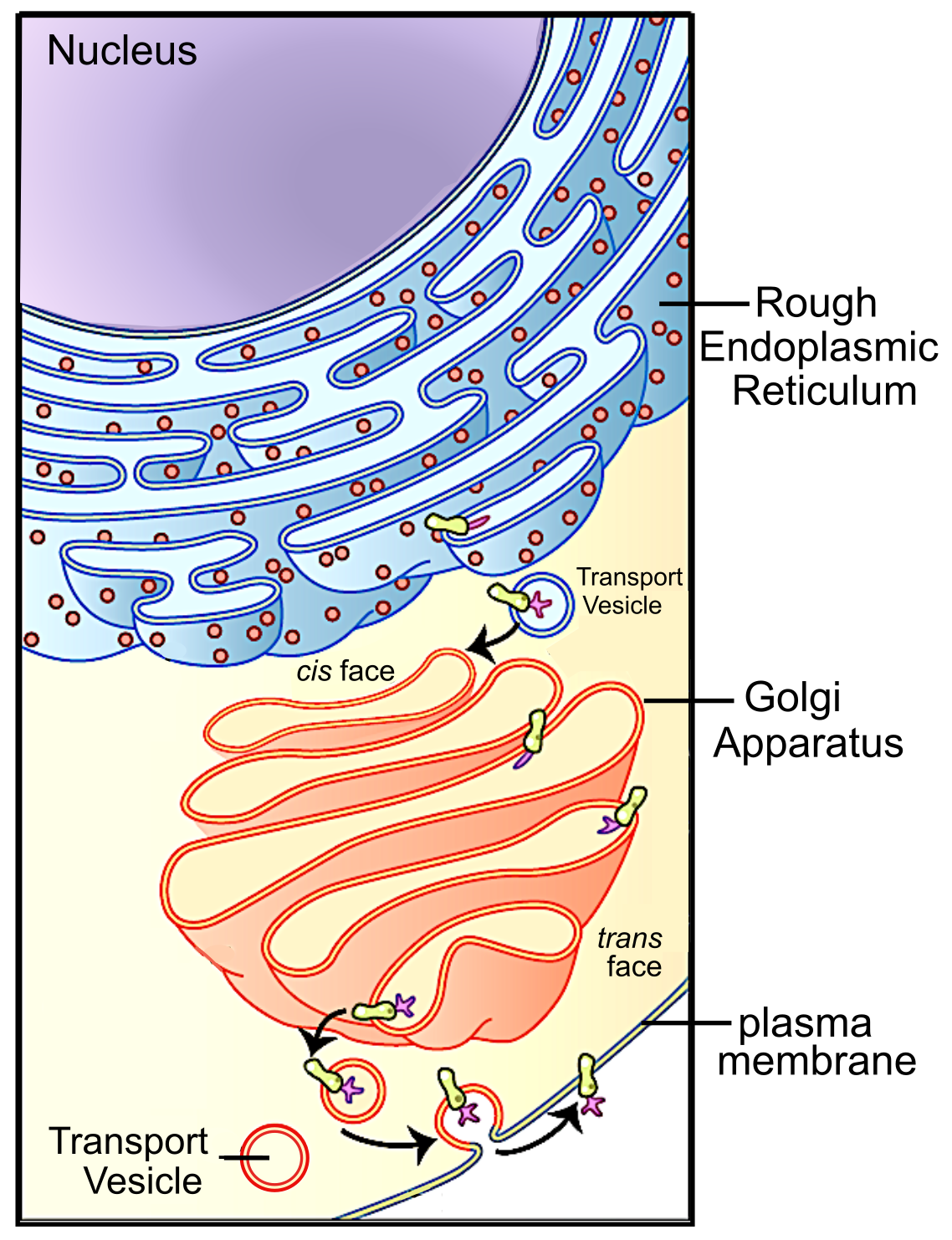
The Endoplasmic Reticulum
The endoplasmic reticulum (ER) is a series of interconnected membranous sacs and tubules that collectively modifies proteins and synthesizes lipids. However, these two functions take place in separate areas of the ER: the rough ER and the smooth ER, respectively.
We call the ER tubules’ hollow portion the lumen or cisternal space. The ER’s membrane, which is a phospholipid bilayer embedded with proteins, is continuous with the nuclear envelope.
Rough ER
Scientists have named the rough endoplasmic reticulum as such because the ribosomes attached to its cytoplasmic surface give it a studded appearance when viewing it through an electron microscope.
Ribosomes transfer their newly synthesized proteins into the rough endoplasmic reticulum’s lumen (interior) where they undergo structural modifications, such as folding or acquiring side chains. These modified proteins incorporate into cellular membranes—the endoplasmic reticulum’s or that of other organelles’ membranes. The proteins can also be secreted from the cell (such as protein hormones and enzymes). The cytoplasmic side of the rough endoplasmic reticulum also makes phospholipids for cellular membranes.
If the phospholipids or modified proteins are not destined to stay in the rough endoplasmic reticulum, they will reach their destinations via transport vesicles that bud from the reticulum’s membrane.
Since it is engaged in modifying proteins (such as enzymes, for example) that secrete from the cell, you would be correct in assuming that the rough endoplasmic reticulum is abundant in cells that secrete proteins. This is the case with liver cells, for example.
Smooth ER
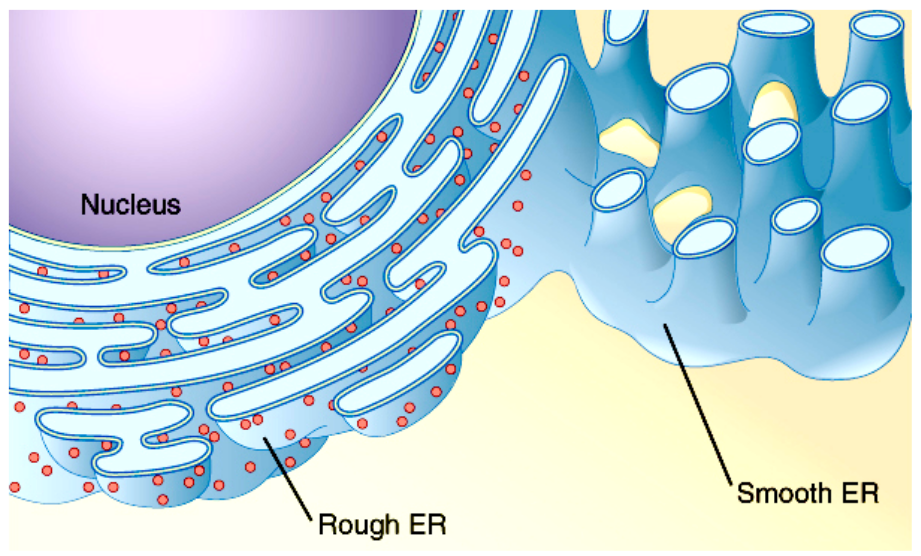
The smooth endoplasmic reticulum is continuous with the rough endoplasmic reticulum but has few or no ribosomes on its cytoplasmic surface. Smooth endoplasmic reticulum functions include the synthesis of carbohydrates, lipids, and steroid hormones; detoxification of medications and poisons; and storing calcium ions.
The Golgi Apparatus
We have already mentioned that vesicles can bud from the endoplasmic reticulum and transport their contents elsewhere, but where do the vesicles go? Before reaching their final destination, the lipids or proteins within the transport vesicles still need sorting, packaging, and tagging so that they end up in the right place. Sorting, tagging, packaging, and distributing lipids and proteins takes place in the Golgi apparatus (also called the Golgi body), a series of flattened membranous sacs.
The side of the Golgi apparatus that is closer to the endoplasmic reticulum is called the cis face. The opposite side is the trans face. The transport vesicles that formed from the endoplasmic reticulum travel to the cis face, fuse with it, and empty their contents into the Golgi apparatus’ lumen. As the proteins and lipids travel through the Golgi, they undergo further modifications that allow them to be sorted. The most frequent modification is adding short sugar molecule chains. These newly modified proteins and lipids then tag with phosphate groups or other small molecules in order to travel to their proper destinations.
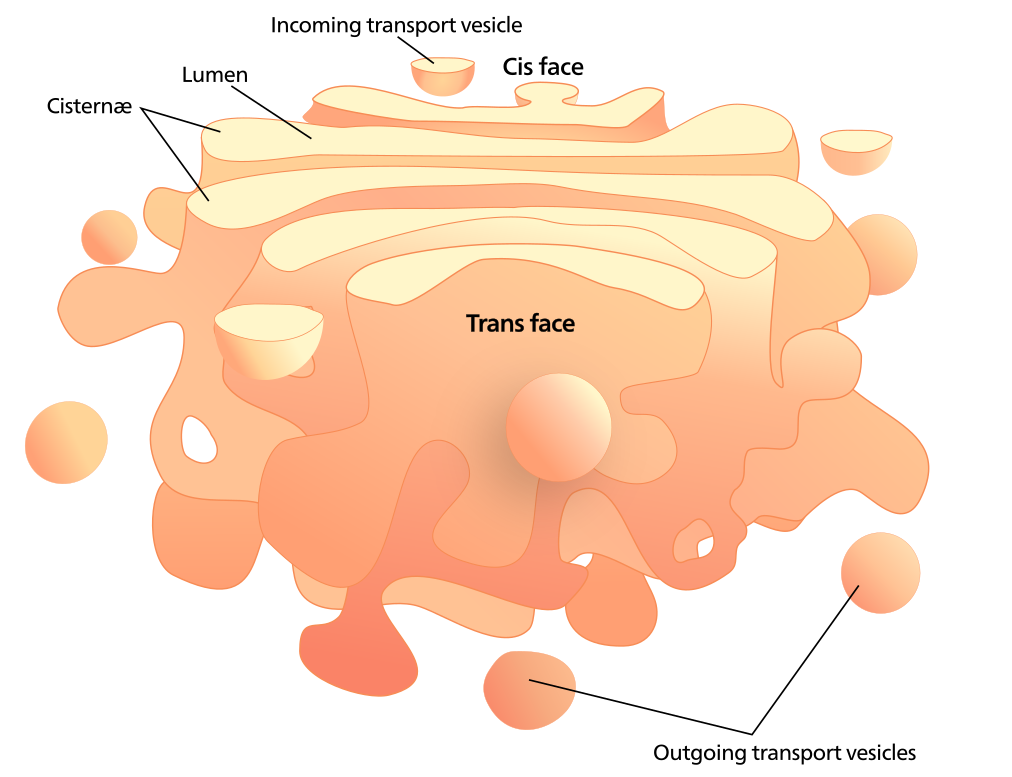
Finally, the modified and tagged proteins are packaged into secretory vesicles that bud from the Golgi’s trans face. While some of these vesicles deposit their contents into other cell parts where they will be used, other secretory vesicles fuse with the plasma membrane and release their contents outside the cell.
In another example of form following function, cells that engage in a great deal of secretory activity (such as salivary gland cells that secrete digestive enzymes or immune system cells that secrete antibodies) have an abundance of Golgi.
Vesicles and Vacuoles
Vesicles and vacuoles are membrane-bound sacs that function in storage and transport. Other than the fact that vacuoles are somewhat larger than vesicles, there is a very subtle distinction between them. Vesicle membranes can fuse with either the plasma membrane or other membrane systems within the cell. Additionally, some agents such as enzymes within plant vacuoles break down macromolecules. The vacuole’s membrane does not fuse with the membranes of other cellular components.
Lysosomes
Animal cells have another set of organelles that most plant cells do not: lysosomes. The lysosomes are the cell’s “garbage disposal” and “recycling centers.” Enzymes within the lysosomes aid in breaking down proteins, polysaccharides, lipids, nucleic acids, and even worn-out organelles. These enzymes are active at a much lower pH than the cytoplasm’s pH. Therefore, the pH within lysosomes is more acidic than the cytoplasm’s pH. Many reactions that take place in the cytoplasm could not occur at a low pH, so again, the advantage of compartmentalizing the eukaryotic cell into organelles is apparent.
Lysosomes also use their hydrolytic enzymes to destroy pathogens (disease-causing organisms) that might enter the cell. A good example of this occurs in macrophages, a group of white blood cells that are part of your body’s immune system. In a process that scientists call phagocytosis or endocytosis, a section of the macrophage’s plasma membrane invaginates (folds in) and engulfs a pathogen. The invaginated section, with the pathogen inside, then pinches itself off from the plasma membrane and becomes a vesicle. The vesicle fuses with a lysosome. The lysosome’s hydrolytic enzymes then destroy the pathogen.
Peroxisomes
Peroxisomes were first named for their ability to produce hydrogen peroxide (H2O2), a highly reactive molecule that helps to break down molecules such as uric acid, amino acids, and fatty acids. Peroxisomes also possess the enzyme catalase, which can degrade hydrogen peroxide. Along with the smooth endoplasmic reticulum, peroxisomes also play a role in lipid biosynthesis. Like lysosomes, the compartmentalization of these degradative molecules within an organelle helps protect the cytoplasmic contents from unwanted damage.
Cytoskeleton
If you were to remove all the organelles from a cell, would the plasma membrane and the cytoplasm be the only components left? No. Within the cytoplasm, there would still be ions and organic molecules, plus a network of protein fibers that help maintain the cell’s shape, secure some organelles in specific positions, allow cytoplasm and vesicles to move within the cell, and enable cells within multicellular organisms to move. Collectively, scientists call this network of protein fibers the cytoskeleton. There are three types of fibers within the cytoskeleton: microfilaments, intermediate filaments, and microtubules. Microfilaments thicken the cortex around the cell’s inner edge. Like rubber bands, they resist tension. There are microtubules in the cell’s interior where they maintain their shape by resisting compressive forces. There are intermediate filaments throughout the cell that hold organelles in place.
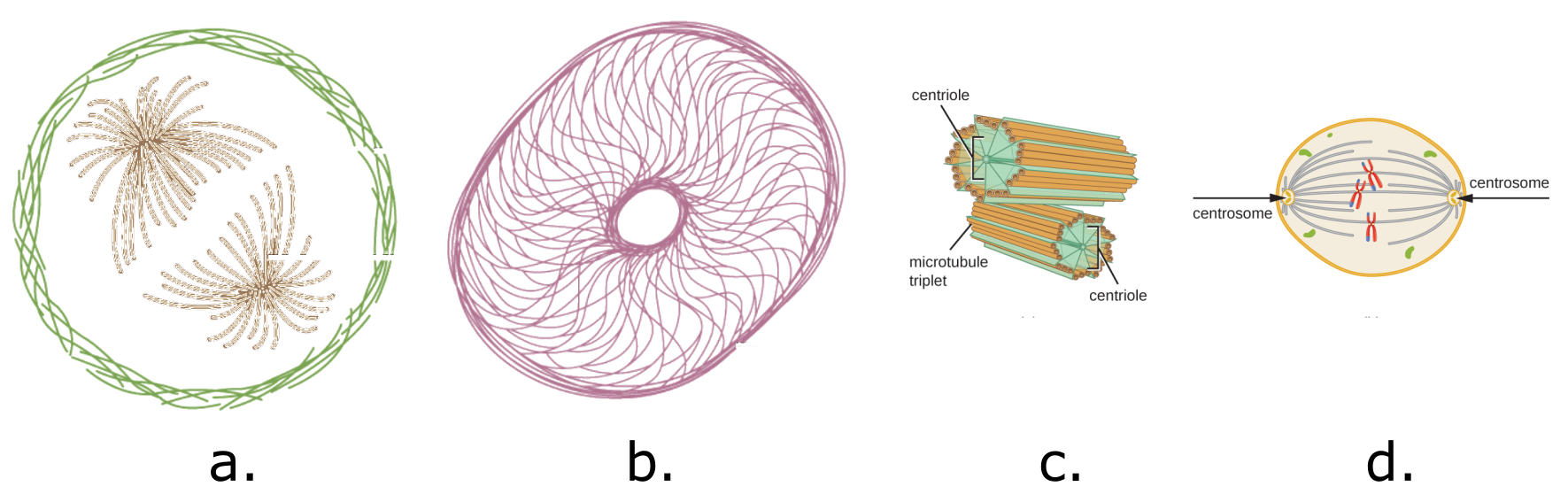
The cytoskeleton is both a scaffold for the cell structure and a framework for many cellular activities, including movement and cell division. In our factory analogy, the cytoskeleton is represented by structural beams, as well as any transport infrastructure, like hallways, elevator tracks, or even small railroads. In the cell, the cytoskeleton is made up of many different protein filaments. Parts of the cytoskeleton actually move other organelles around the cell or change the shape of the cell. When a muscle cell contracts or shortens, it uses the microfilaments made up of the proteins actin and myosin. One special organelle composed of microtubules is located near the nucleus, the centrosome. The centrosome contains a pair of microtubule bundles known as centrioles. Centrioles are important because they move chromosomes to opposite ends of the cell during cell replication (mitosis). Nerve cells (neurons) do not have centrioles and so cannot replicate. Microtubules also form appendages such as the flagellum of the sperm cell and the cilia of the cells of the respiratory system.
Mitochondria
Scientists often call mitochondria (singular = mitochondrion) “powerhouses” or “energy factories” of both plant and animal cells because they are responsible for making adenosine triphosphate (ATP), the cell’s main energy-carrying molecule. ATP represents the cell’s short-term stored energy. Cellular respiration is the process of making ATP using the chemical energy in glucose and other nutrients. In mitochondria, this process uses oxygen and produces carbon dioxide as a waste product. In fact, the carbon dioxide that you exhale with every breath comes from the cellular reactions that produce carbon dioxide as a byproduct.
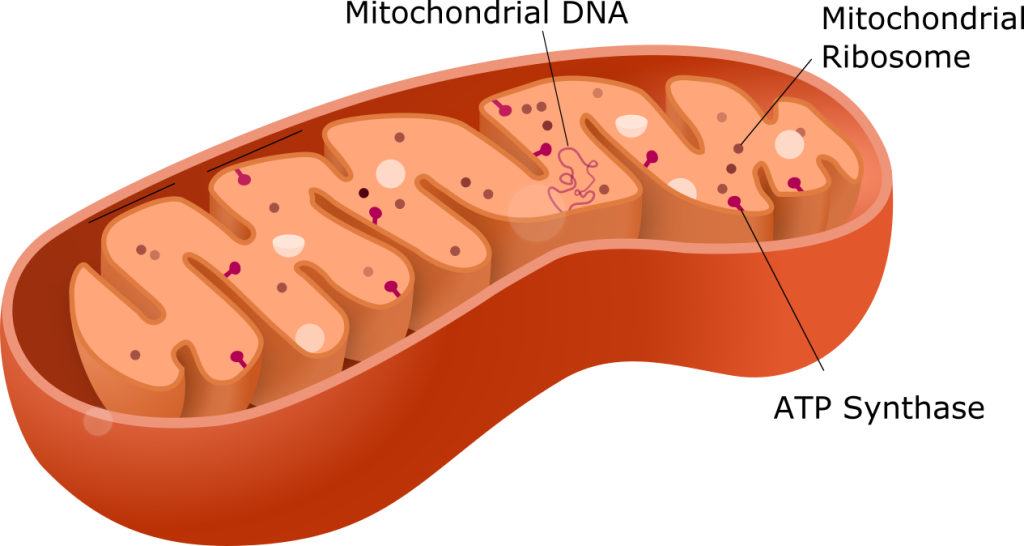
In keeping with our theme of form following function, it is important to point out that muscle cells have a very high concentration of mitochondria that produce ATP. Your muscle cells need considerable energy to keep your body moving. When your cells don’t get enough oxygen, they do not make much ATP. Instead, producing lactic acid accompanies the small amount of ATP they make in the absence of oxygen.
Mitochondria are oval-shaped, double-membrane organelles. Each membrane is a phospholipid bilayer embedded with proteins. The inner layer has folds called cristae. We call the area surrounded by the folds the mitochondrial matrix. The cristae and the matrix have different roles in cellular respiration. Mitochondria have their own DNA, termed mitochondrial DNA (mtDNA), and can replicate independently of the cell.
Section Summary
The nucleus is the control center of the cell, and it stores the DNA, which contains the instructions for how to build all the protein products required by the cell. The nucleus is like the factory command center, which stores the instructions needed to build its product. A single molecule of DNA wound around specialized proteins is referred to as chromatin. Chromosomes are very condensed forms of chromatin and are like the different books in a library.
The nucleus is surrounded by a double-layered membrane called the nuclear envelope. The nuclear envelope is studded with nuclear pores that allow information from inside the nucleus to enter the cytoplasm. Imagine a factory command center. It would not be very effective if it did not have doors or windows through which to pass information to the rest of the factory.
There are several important structures found within the nucleus. The most visible of these is the nucleolus. In contrast to other organelles, the nucleolus is not bound by a membrane. Instead, it is an aggregate of molecules where ribosomes, another type of non-membranous organelle, are assembled. Ribosomes are amazing nanoscopic structures that are responsible for taking a genetic “blueprint” and using it to build a protein. (Nanoscopic means one thousand times smaller than microscopic!) Think of ribosomes as small versatile assembly machines.
Most organelles, including ribosomes (after they are built in the nucleus), are found in the cytoplasm, which is the substance found between the nucleus and the cell membrane (number eight in the cell factory diagram). The cytoplasm is analogous to a factory floor, where all the work takes place.
All this work requires energy. Most factories need some sort of power plant that converts fuel into a form of energy that can be captured to do work. (Work is defined as a change in the form or position of some object.) In the cell, this job is accomplished by organelles called mitochondria (the singular form is mitochondrion). This organelle plays a critical role in harvesting chemical energy for the cell. Mitochondria harvest the energy stored in monosaccharides (and other organic monomers) and are the organelles responsible for providing energy for all cellular functions in animal cells.
Factories often have assembly lines that put together the company’s product. In the cell, proteins and other cellular components are put together, or assembled, by the endoplasmic reticulum, a series of sacs and tubes. In eukaryotes, ribosomes are associated with the rough endoplasmic reticulum, which gets its name from the beaded appearance that the ribosomes give it. The smooth endoplasmic reticulum (without ribosomes) can have different functions depending on the cell type, but it is often the site for the synthesis of lipids.
The Golgi apparatus is the packaging and shipping center of the cell, where the proteins that were built by the endoplasmic reticulum assembly line are delivered to different parts of the cell, or in multicellular organisms, to different parts of the body. Often the Golgi apparatus packages proteins in vesicles and vacuoles, which are membrane-bound sacs that function in storage and transport. Vesicles are specialized for transport and some other functions. Their membranes can fuse with the plasma membrane, allowing them to empty their contents into the extracellular space. Vesicles also may fuse with the membranes of the endoplasmic reticulum and Golgi apparatus, allowing them to empty their contents into those organelles. Lysosomes are specialized vesicles found only in animal cells. Lysosomes contain powerful digestive enzymes that can recycle cellular parts or destroy external invaders. Peroxisomes metabolize some types of metabolic waste, producing hydrogen peroxide (H2O2) as a result. Vacuoles are specialized mainly for storage. Their membranes do not fuse with the membranes of other cellular components.
While most animal cells have the structures shown above, variations occur depending on the function of the cell. Think about cars — while most cars have the same parts, there will be differences between a sedan and an off-road vehicle. Among animal cells, extreme examples of specialization include red blood cells (containers of hemoglobin to transport oxygen) and nerve cells (dedicated to transmission and integration of signals (see Figure 6.19). The hundreds of cell types in the body differ in the arrangement and number of organelles, along with differing in their physical structure.
Learn By Doing 6.4
The rough ER has its name due to what associated structures?
- Golgi apparatus
- ribosomes
- lysosomes
- proteins
Which of the following is a function of the rough ER?
- production of proteins
- detoxification of certain substances
- synthesis of steroid hormones
- regulation of intracellular calcium concentration
Which of the following is a feature common to all three components of the cytoskeleton?
- They all serve to scaffold the organelles within the cell.
- They are all characterized by roughly the same diameter.
- They are all polymers of protein subunits.
- They all help the cell resist compression and tension.
Which of the following organelles produces large quantities of ATP when both glucose and oxygen are available to the cell?
- mitochondria
- peroxisomes
- lysosomes
- ER
Which of the following structures could be found within the nucleolus?
- chromatin
- histones
- ribosomes
- nucleosomes
Explain how the different structures of the ER, mitochondria, and Golgi apparatus support their differing functions.
What is the function of the ribosome?
- The ribosome is the site for all chemical reactions in the cell.
- The ribosome makes energy that is usable for the cell.
- The ribosome builds proteins using the information contained in DNA.
- The ribosome provides the information contained in DNA.
What structure stores genetic information?
- cytoplasm
- mitochondria
- nucleus
- chromosome/DNA
ATP in Living Systems
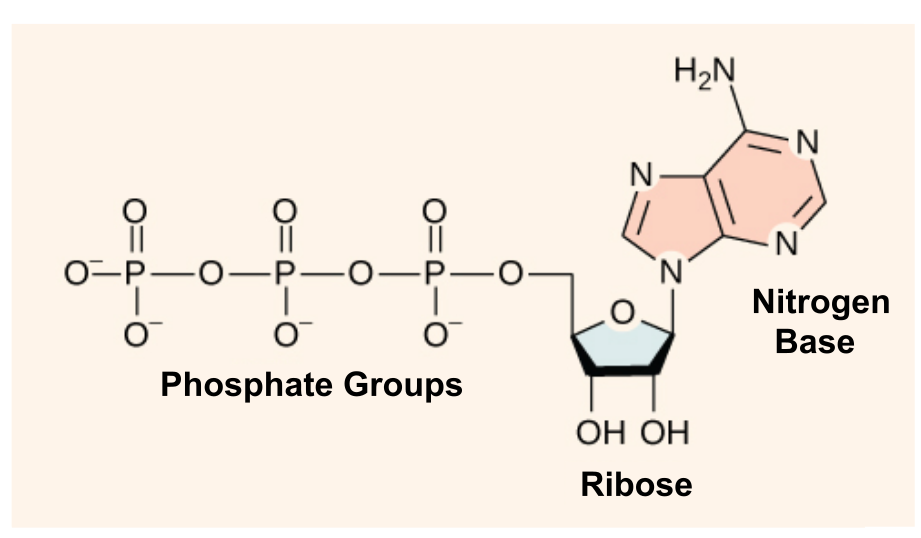
At the heart of ATP is a molecule of adenosine monophosphate (AMP), which is composed of an adenine molecule bonded to both a ribose molecule and a single phosphate group. Ribose is a five-carbon sugar found in RNA, and AMP is one of the nucleotides in RNA. The addition of a second phosphate group to this core molecule results in adenosine diphosphate (ADP); the addition of a third phosphate group forms adenosine triphosphate (ATP).
The ATP-ADP Cycle
A living cell cannot store significant amounts of free energy. Excess free energy would result in an increase of heat in the cell, which would denature enzymes and other proteins and thus destroy the cell. Rather, a cell must be able to store energy safely and release it for use only as needed. Living cells accomplish this using ATP, which can be used to fill any energy need of the cell. How? ATP functions as a rechargeable battery.
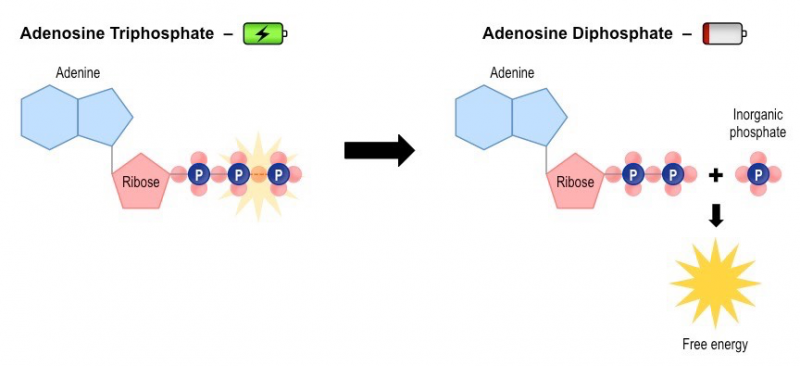
Phosphate groups are negatively charged and thus repel one another when they are arranged in series, as they are in ADP and ATP. This repulsion makes the ADP and ATP molecules inherently unstable. The release of one or two phosphate groups from ATP releases energy to do work in the cell. ATP hydrolysis occurs when water is added to break the chemical bond and is the opposite of a dehydration synthesis reaction (see Figure 6.20). When ATP is broken down, usually by the removal of its terminal phosphate group, energy is released. This free energy is used to do work by the cell, usually by the binding of the released phosphate to another molecule, thus activating it. For example, in the mechanical work of muscle contraction, ATP supplies energy to move the contractile muscle proteins. ATP hydrolysis occurs throughout the cell, wherever energy is needed.
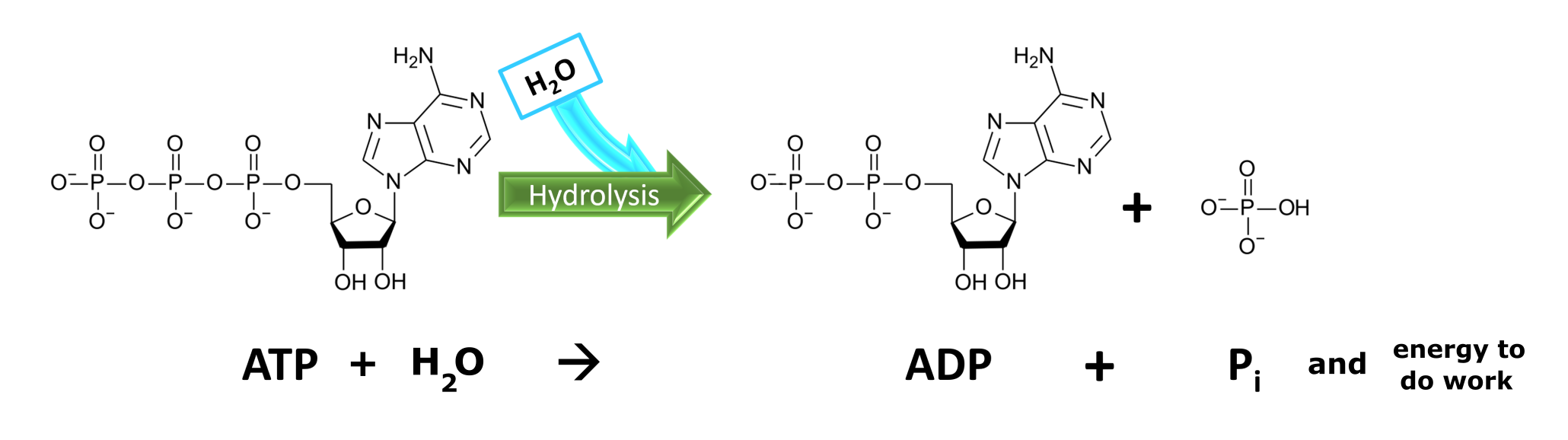
As will be discussed below, the energy used to “recharge” ATP comes from the bond energy contained in our food. The process is called aerobic cellular respiration and occurs primarily in mitochondria. If ATP hydrolysis is the energy-releasing ATP to ADP half of a cycle, cellular respiration can be expressed as the energy-requiring process of converting ADP and inorganic phosphate to ATP.
Learn By Doing 6.5
Energy is stored long-term in the bonds of _____. Energy used to perform work comes most directly from _____ molecule.
- ATP, glucose
- an anabolic molecule, a catabolic molecule
- glucose, ATP
- a catabolic molecule, an anabolic molecule
The energy currency used by cells is _____.
- ATP
- ADP
- AMP
- adenosine
Aerobic Cellular Respiration
ATP functions as the energy currency for cells. It allows cells to store energy briefly and transport it within themselves to support chemical reactions that require a significant investment of energy. ATP hydrolysis releases energy used to do work in the cell. During the process, a phosphate group is detached using a water molecule, and ADP and inorganic phosphate (Pi) are produced. Energy derived from glucose and oxygen catabolism is used to convert the resulting ADP and inorganic phosphate back into ATP in a process called aerobic cellular respiration. The summary equation is shown in Figure 6.21.
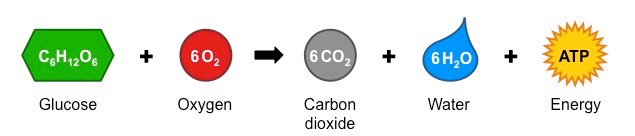
This process typically includes three steps: glycolysis, the citric acid cycle, and the electron transport chain. As you read, note the cellular location, the number of ADP molecules recharged to ATP, and the oxygen requirement of each process. All the stages of this process are summarized in Figure 6.24.
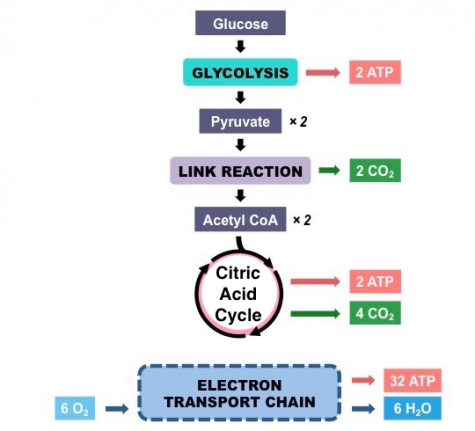
Glycolysis
You have read that nearly all of the energy used by living things comes in the form of bonds within glucose. Glycolysis is the first step in the breakdown of glucose to extract energy for cell metabolism. Many living organisms carry out glycolysis as part of their metabolism. Glycolysis takes place in the cytoplasm of human cells and does not require oxygen.
Glycolysis begins with the six-carbon, ring-shaped structure of a single glucose molecule and ends with two molecules of a three-carbon sugar called pyruvate. If the cell cannot catabolize the pyruvate molecules further, it will harvest only two ATP molecules from one molecule of glucose. For example, mature red blood cells are only capable of glycolysis, which is their sole source of ATP. If glycolysis is interrupted, these cells eventually die.
Citric Acid Cycle
In our cells, the pyruvate molecules produced at the end of glycolysis are transported into mitochondria, which are sites of aerobic cellular respiration. If oxygen is available, aerobic respiration will go forward. Part of the Citric Acid Cycle is considered an aerobic pathway (oxygen-requiring) because some of the intermediate molecules produced must transfer their electrons to the next pathway in the system (the Electron Transport Chain), which needs oxygen to function. If oxygen is not present, this transfer does not occur.
Electron Transport Chain
You have just read about two pathways in glucose catabolism—glycolysis and the citric acid cycle—that generate ATP. Most of the ATP generated during the aerobic catabolism of glucose, however, is not generated directly from these pathways. Rather, it comes from a process that begins with passing electrons through a series of chemical reactions to a final electron acceptor, oxygen. These reactions take place in specialized protein complexes located in the inner membrane of the mitochondria. The energy of the electrons is harvested and used to generate an electrochemical gradient of hydrogen ions across the inner mitochondrial membrane. The potential energy of this gradient is used to generate ATP by providing the energy to add phosphate groups to ADP molecules. The entirety of this process is called oxidative phosphorylation, as oxygen is required as the terminal electron acceptor, and phosphate groups are added to ADP molecules.
The electron transport chain is the last part of aerobic respiration and is the only part of metabolism that uses atmospheric oxygen. In animals, oxygen enters the body through the respiratory system. Electron transport is a series of chemical reactions that resembles a bucket brigade in that electrons are passed rapidly from one part to the next, to the endpoint of the chain where oxygen is the final electron acceptor and water is produced. If there were no oxygen present in the mitochondrion, the electrons could not be removed from the system, and the entire electron transport chain would back up and stop. The mitochondria would be unable to generate new ATP in this way, and the cell would ultimately die from a lack of energy. This is the reason we must breathe to draw in new oxygen.
ATP Yield
The number of ATP molecules generated from the catabolism of glucose varies but is roughly 32 ATP. Remember that two ATP were produced directly in both glycolysis and the citric acid cycle, resulting in a total yield of 36 ATP per glucose. This represents an efficiency of approximately 35%, with the remaining energy potential lost as heat or other products.
Learn By Doing 6.6
For each of the three parts of aerobic cellular respiration, describe where they take place and how much ATP is generated.
Using your new-found understanding of aerobic cellular respiration, explain why suffocation causes death.
Cyanide inhibits cytochrome c oxidase, part of the electron transport chain. If cyanide poisoning occurs, what do you expect the effect to be on ATP synthesis?
Anaerobic Respiration
Learning Objective
Fermentation
In aerobic respiration, the final electron acceptor is an oxygen molecule, O2. If aerobic respiration occurs, then ATP will be produced using the energy of the high-energy electrons carried by the electron transport chain. If aerobic respiration does not occur, the electron carriers must be altered so that they can be used in glycolysis. How is this done? Humans use an organic molecule (pyruvate/pyruvic acid) as the final electron acceptor. Processes that use an organic molecule to alter the electron carriers are collectively referred to as fermentation.
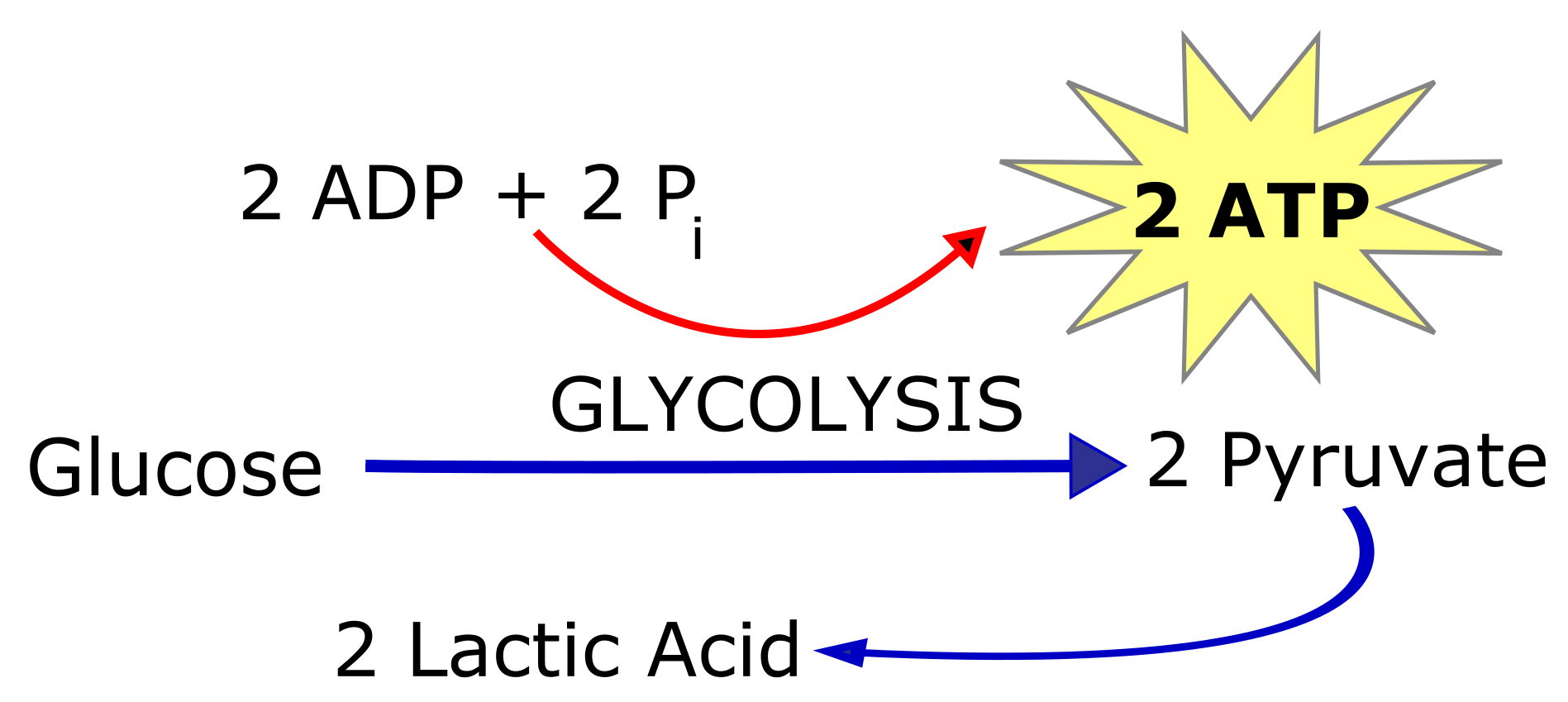
Lactic Acid Fermentation
The fermentation method used by animals (and some bacteria like those in yogurt) is lactic acid fermentation. This occurs routinely in mammalian red blood cells and in skeletal muscle that has insufficient oxygen supply to allow aerobic respiration to continue (that is, in muscles used to the point of fatigue). In muscles, lactic acid produced by fermentation must be removed by the blood circulation and brought to the liver for further metabolism.
Learn By Doing 6.7
Describe an important difference between aerobic and anaerobic respiration other than the need for oxygen.
Connections between Cells and Cellular Activities
You may already know that tissue is a group of similar cells working together. As you might expect, if cells are to work together, they must communicate with each other, just as you need to communicate with others if you work on a group project. Let’s take a look at how cells communicate with each other.
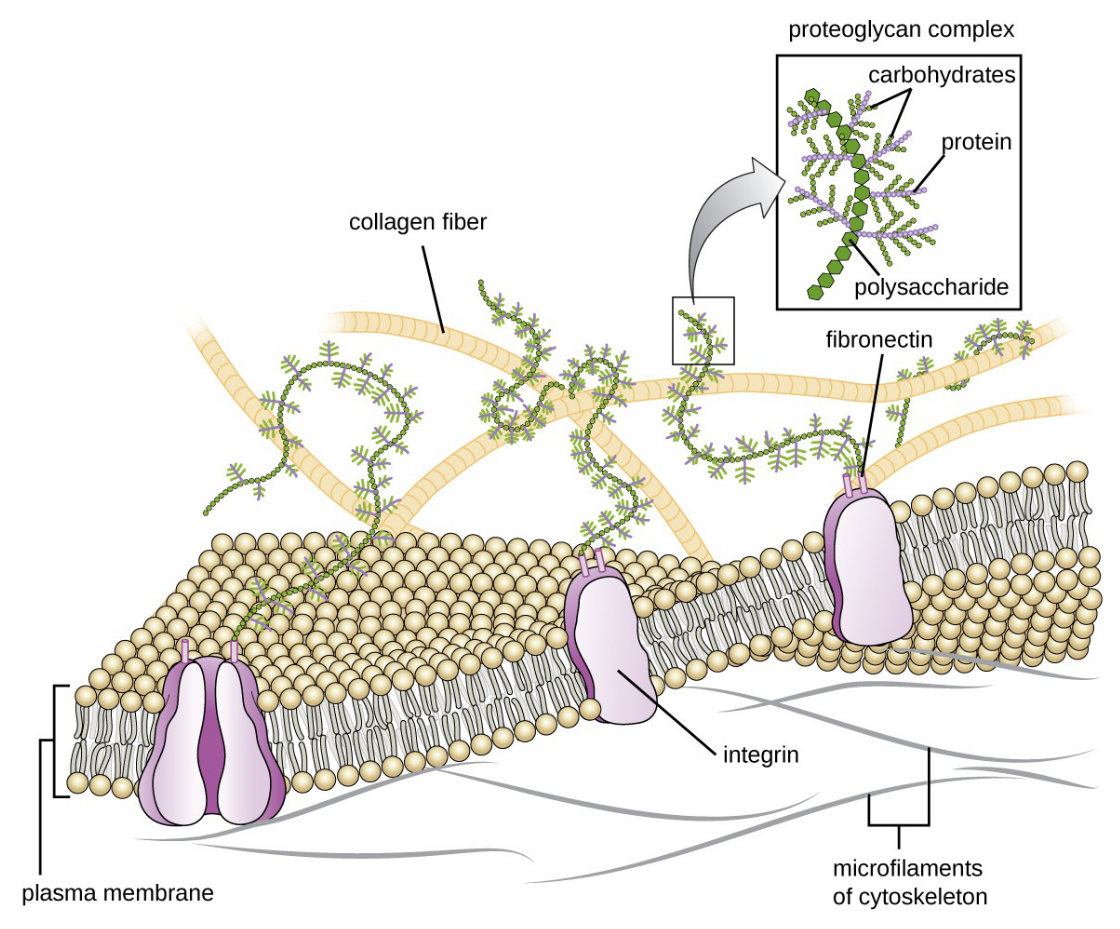
While cells in most multicellular organisms release materials into the extracellular space, animal cells will be discussed as an example. The primary components of these materials are proteins, and the most abundant protein is collagen. Collagen fibers are interwoven with proteoglycans, which are carbohydrate-containing protein molecules. Collectively, we call these materials the extracellular matrix. Not only does the extracellular matrix hold the cells together to form a tissue, but it also allows the cells within the tissue to communicate with each other. How can this happen?
The extracellular matrix consists of a network of proteins and carbohydrates. Cells have protein receptors on their plasma membranes’ extracellular surfaces. When a molecule within the matrix binds to the receptor, it changes the receptor’s molecular structure. The receptor, in turn, changes the microfilaments’ conformation positioned just inside the plasma membrane. These conformational changes induce chemical signals inside the cell that reach the nucleus and turn “on” or “off” the transcription of specific DNA sections, which affects the associated protein production, thus changing the activities within the cell.
Blood clotting provides an example of the extracellular matrix’s role in cell communication. When the cells lining a blood vessel are damaged, they display a protein receptor, which we call tissue factor. When tissue factor binds with another factor in the extracellular matrix, it causes platelets to adhere to the damaged blood vessel’s wall, stimulates the adjacent smooth muscle cells in the blood vessel to contract (thus constricting the blood vessel), and initiates a series of steps that stimulate the platelets to produce clotting factors.
Intercellular Junctions
Cells can also communicate with each other via direct contact or intercellular junctions. Animal cell contacts include tight junctions, desmosomes, and gap junctions.
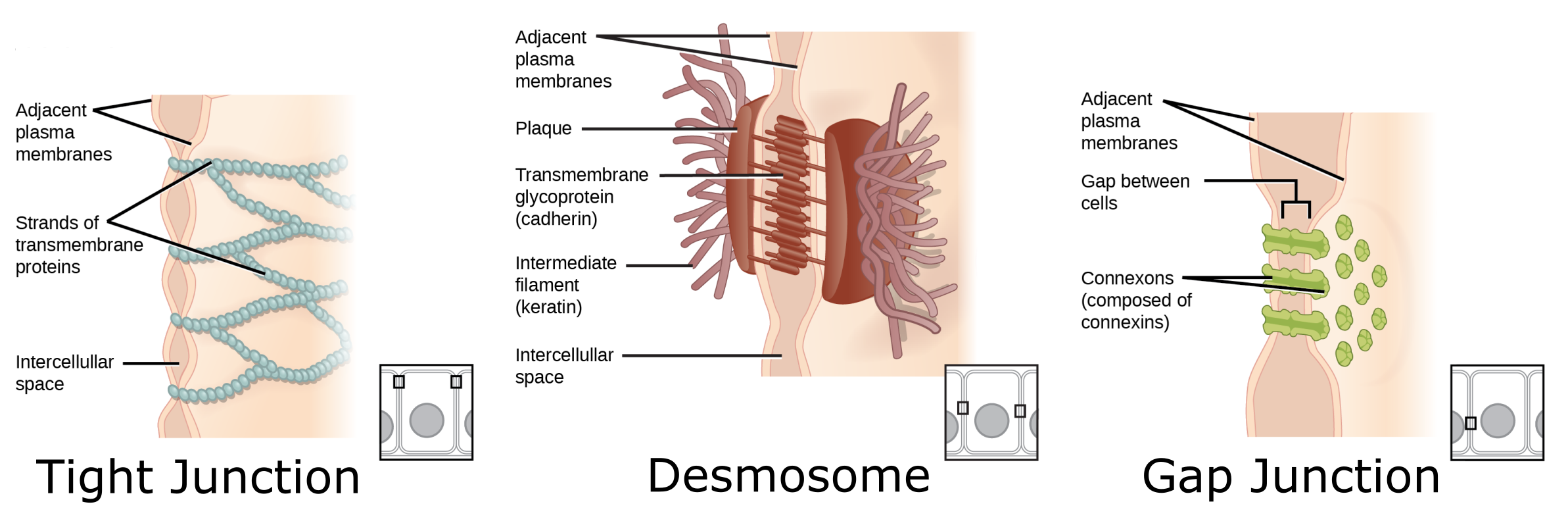
A tight junction is a watertight seal between two adjacent animal cells. Proteins (predominantly two proteins called claudins and occludins) tightly hold the cells against each other. This tight adherence prevents materials from leaking between the cells; tight junctions are typically found in epithelial tissues that line internal organs and cavities and comprise most of the skin. For example, the tight junctions of the epithelial cells lining your urinary bladder prevent urine from leaking out into the extracellular space.
Desmosomes, also called adhesive junctions, act like spot welds between adjacent epithelial cells. Cadherins, short proteins in the plasma membrane, connect to intermediate filaments to create desmosomes. The cadherins connect two adjacent cells and maintain the cells in a sheet-like formation in organs and tissues that stretch, like the skin, heart, and muscles.
Gap junctions in animal cells are channels between adjacent cells that allow for transporting ions, nutrients, and other substances that enable cells to communicate. Gap junctions develop when a set of six proteins (connexins) in the plasma membrane arrange themselves in an elongated donut-like configuration – a connexon. When the connexon’s pores (“doughnut holes”) in adjacent animal cells align, a channel between the two cells forms. Gap junctions are particularly important in cardiac muscle. The electrical signal for the muscle to contract passes efficiently through gap junctions, allowing the heart muscle cells to contract in tandem.
Learn By Doing 6.8
Type your exercises here.
Cell Division and Control of Cell Number
Learning Objectives:
- Describe somatic cell division.
- Describe how cell number is maintained and the processes associated with cell death: apoptosis and necrosis.
Even though we may not feel any changes from day to day, we are constantly repairing and replacing cells within our bodies. It’s estimated that approximately 300 million cells die every minute in our body! These cells must be replaced by identical functional cells for us to survive and maintain homeostasis. The process of cell proliferation or cell division is termed mitosis. Mitosis produces two daughter cells that are identical (clones) to the parent cell.
Cell division is a carefully regulated process. There are “checkpoints” between phases, in which the cell “self-checks.” Through molecular interactions, the cell makes sure that it is ready to divide; for example, it checks to see that its DNA has not been damaged. If all criteria are met, the cell moves forward through the cell cycle. Normal cells usually divide only a limited number of times and usually do so when stimulated by certain molecular signals. For example, if you move to a higher altitude, your body will produce more red blood cells in order to supply enough oxygen despite the thinner air.
Mitosis is also regulated by genes. Some genes become active to stimulate mitosis, and other genes act as the brakes to turn it off (suppressor genes). If our body loses control over mitosis, the result would be the production of too many (hyperplasia) abnormal cells and would form a tumor. If the tumor is encapsulated, it is termed benign. If not, the mutated cells can spread, which is known as cancer. Cancer cells, on the other hand, have lost the normal cell cycle controls and therefore can divide indefinitely.
When a cell is performing its normal day-to-day functions, it is in a state termed interphase. When it is time for that cell to replicate, genes become active and stimulate it to begin mitosis. Mitosis is characterized by four phases. Remember that if a cell is going to form 2 new identical cells, it must first replicate its DNA and organelles. It is during interphase that the DNA is replicated to form 2 identical strands. These strands of identical DNA are termed chromatids and are held together with a centromere. The stages of mitosis occur in sequence with specific events in each one. It is only during mitosis that chromosomes are visible. Usually, DNA is in its threadlike chromatin form.
Apoptosis
How does a single fertilized cell develop into something as marvelous and intricate as the human body? Part of the answer is apoptosis or programmed cell death. For example, the early hand of an embryo is a stubby appendage. As cells between the fingers selectively die off, they sculpt the hand, leaving behind the separate fingers. In adults, apoptosis is one way that tissues maintain their integrity. Cells selectively die off to allow the renewal of tissues such as the stomach, lungs, liver, and so on. If apoptosis ceases in the tissue’s cells, a tumor can form, perhaps becoming cancerous. Apoptosis is distinguished from necrosis, which is cell death as a result of injury. Much like proliferation, there is a series of tightly regulated events that lead to apoptosis. Apoptosis is a cellular program that is internally determined, and billions of cells die this way every day. However, external trauma, toxins, or infection cause a different type of cell death called necrosis. Even though both mechanisms lead to dead cells, apoptosis is a program to maintain cell number whereas necrosis is a consequence of intense cell damage.
Homeostatic Imbalances in Cell Division
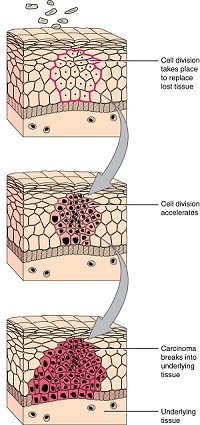
Cancer is a generic term for many diseases where cells escape regulatory signals. Uncontrolled growth, invasion into adjacent tissues, and colonization of other organs, if not treated early enough, are its hallmarks. Health suffers when tumors “rob” blood supply from the “normal” organs.
A mutation is defined as a permanent change in the DNA of a cell. Epigenetic modifications, changes that do not affect the code of the DNA but alter how the DNA is decoded, are also known to generate abnormal cells. Alterations in the genetic material may be caused by environmental agents, infectious agents, or errors in the replication of DNA. They accumulate with age. Many mutations do not cause any noticeable change in the functions of a cell. However, if the modification affects key proteins that have an impact on the cell’s ability to proliferate in an orderly fashion, the cell starts to divide abnormally. As changes in cells accumulate, they lose their ability to form regular tissues. A tumor, a mass of cells displaying abnormal architecture, forms in the tissue. Many tumors are benign, meaning they do not metastasize or cause disease. A tumor becomes malignant, or cancerous, when it breaches the confines of its tissue, promotes angiogenesis, attracts the growth of capillaries, and metastasizes to other organs. The specific names of cancers reflect the tissue of origin. Cancers derived from epithelial cells are referred to as carcinomas. Cancer in myeloid tissue or blood cells forms myelomas. Leukemias are cancers of white blood cells, whereas sarcomas derive from connective tissue. Cells in tumors differ both in structure and function. Some cells, called cancer stem cells, appear to be a subtype of cell responsible for uncontrolled growth. Recent research shows that contrary to what was previously assumed, tumors are not disorganized masses of cells, but have their own structures.
Cancer treatments vary depending on the disease’s type and stage. Traditional approaches, including surgery, radiation, chemotherapy, and hormonal therapy, aim to remove or kill rapidly dividing cancer cells, but these strategies have their limitations. Depending on a tumor’s location, for example, cancer surgeons may be unable to remove it. Radiation and chemotherapy are difficult, and it is often impossible to target only the cancer cells. The treatments inevitably destroy healthy tissue as well. To address this, researchers are working on pharmaceuticals that can target specific proteins implicated in cancer-associated molecular pathways.
Learn By Doing 6.9
Type your exercises here.
*
“Learn By Doing” and “Did I Get This?” Feedback
Learn By Doing 6.1
True or false: Cells are alive.
Correct. Cells are alive and are complex structures.
The human body contains bacterial cells and human cells. In fact, bacterial cells outnumber human cells 10 to one. Both are considered cells because they:
Hint: Think about which choice fits into cell theory.
- have membranes
Incorrect. There are structures called micelles that are surrounded by membrane but are not cells. This is why the presence of membranes is NOT a part of cell theory. - can divide into new cells
Correct. The ability to divide into new cells is one of the tenets of cell theory. - are composed of biomolecules
Incorrect. While bacterial and human cells are both composed of biomolecules, there are many things that are made of biomolecules that are not cellular, like enzymes. This is why being made of biomolecules is NOT part of cell theory.
What does the cell theory propose?
- All organisms are made of one or more cells.
- All the life functions of an organism occur within cells.
- All cells come from existing cells.
- All of the above
Learn By Doing 6.2
Which of these do all prokaryotes and eukaryotes share?
- nuclear envelope
- cell walls
- organelles
- plasma membrane
A typical prokaryotic cell __________________ compared to a eukaryotic cell.
- is smaller in size by a factor of 100
- is similar in size
- is smaller in size by a factor of one million
- is larger in size by a factor of 10
Cells are small because as cell size increases, the
- volume and surface area decrease.
- volume increases faster than the surface area.
- surface area and volume increase at the same rate.
- surface area increases faster than the volume.
Antibiotics are medications that are used to fight bacterial infections in humans and other eukaryotes. Consider the following statements; which one is probably true about antibiotics?
- Antibiotics are poisonous to all cells.
- Antibiotics are only poisonous to prokaryotic cells.
- Antibiotics are only poisonous to eukaryotic cells.
You find a cell and examine it under a microscope. You are able to see that the cell has a nucleus. What can you conclude about this cell?
- This cell is a eukaryote.
- You cannot draw conclusions given only this information.
- This cell is a prokaryotic cell.
Which of the following structures is found in BOTH prokaryotes and eukaryotes? (More than one answer is correct.)
- cytoplasm
- ribosome
- nucleus
- DNA
- Mitochondria
Learn By Doing 6.3
The physical characteristics of a cell influence how it functions. For example, the surface area and volume of a cell affect how efficiently waste is removed from the cell via diffusion.
The table below shows data for four different cuboidal cells.
Cell | Length of One Side () |
A | 1 |
B | 2 |
C | 3 |
D | 4 |
Using the information from the table, what is the surface-area-to-volume ratio for the cuboidal cell that removes waste most efficiently?
Hint: The length, width, and height of a cube are all the same.
Calculate the surface area, volume, and surface area to volume ratio for each cell and then compare them.
Cell | Length of One Side () | Total Surface Area (length x width) x # of sides |
Volume (length x width x height) |
Surface Area: Volume Ratio surface area / volume |
A | 1 | (1 x 1) x 6 = 6 | 1 x 1 x 1 = 1 | 6/1 = 6 |
B | 2 | (2 x 2) x 6 = 24 | 2 x 2 x 2 = 8 | 24/8 = 3 |
C | 3 | (3 x 3) x 6 = 54 | 3 x 3 x 3 = 27 | 54/27 = 2 |
D | 4 | (4 x 4) x 6 = 96 | 4 x 4 x 4 = 64 | 96/64 = 1.5 |
The cell that would remove waste most efficiently is cell A. This is because it has the greatest amount of membrane (surface area) for the amount of cytoplasm (volume). The greater the ratio, the better the cell can exchange materials with the environment.
Learn By Doing 6.4
The rough ER has its name due to what associated structures?
- Golgi apparatus
- ribosomes
- lysosomes
- proteins
Which of the following is a function of the rough ER?
- production of proteins
- detoxification of certain substances
- synthesis of steroid hormones
- regulation of intracellular calcium concentration
Which of the following is a feature common to all three components of the cytoskeleton?
- They all serve to scaffold the organelles within the cell.
- They are all characterized by roughly the same diameter.
- They are all polymers of protein subunits.
- They all help the cell resist compression and tension.
Which of the following organelles produces large quantities of ATP when both glucose and oxygen are available to the cell?
- mitochondria
- peroxisomes
- lysosomes
- ER
Which of the following structures could be found within the nucleolus?
- chromatin
- histones
- ribosomes
- nucleosomes
Explain how the different structures of the ER, mitochondria, and Golgi apparatus support their differing functions.
The structure of the Golgi apparatus is suited to its function because it is a series of flattened membranous discs; substances are modified and packaged in sequential steps as they travel from one disc to the next. The structure of Golgi apparatus also involves a receiving face and a sending face, which organize cellular products as they enter and leave the Golgi apparatus. The ER and the mitochondria both have structural specializations that increase their surface area. In the mitochondria, the inner membrane is extensively folded, which increases surface area for ATP production. Likewise, the ER is elaborately wound throughout the cell, increasing its surface area for functions like lipid synthesis, Ca2+storage, and protein synthesis.
What is the function of the ribosome?
- The ribosome is the site for all chemical reactions of the cell.
- The ribosome makes energy that is usable for the cell.
- The ribosome builds proteins using the information contained in DNA.
- The ribosome provides the information contained in DNA.
What structure stores genetic information?
- cytoplasm
- mitochondria
- nucleus
- chromosome/DNA
Learn By Doing 6.5
Energy is stored long-term in the bonds of _____. Energy used to perform work comes most directly from a(n) _____ molecule.
- ATP, glucose
- an anabolic molecule, catabolic molecule
- glucose, ATP
- a catabolic molecule, anabolic molecule
The energy currency used by cells is _____.
- ATP
- ADP
- AMP
- adenosine
Learn By Doing 6.6
For each of the 3 parts of aerobic cellular respiration, describe where they take place and how much ATP is generated.
Process | Location | Net Number of ATP |
Glycolysis | cytoplasm | 2 |
Citric Acid Cycle | mitochondria | 2 |
Electron Transport Chain | mitochondria | 32 |
Using your newfound understanding of aerobic cellular respiration, explain why suffocation causes death.
Our Answer: The electron transport chain provides the majority of our ATP and this process requires oxygen. Suffocation deprives us of oxygen. No oxygen = much less ATP = insufficient energy for cell function = death.
Cyanide inhibits cytochrome c oxidase, part of the electron transport chain. If cyanide poisoning occurs, what do you expect the effect to be on ATP synthesis?
Our Answer: The electron transport chain provides the majority of our ATP. Cyanide interferes with this process by disabling an important enzyme. Cyanide = NO cytochrome c oxidase function = much less ATP = insufficient energy for cell function = death.
Learn By Doing 6.7
Describe an important difference between aerobic and anaerobic respiration other than the need for oxygen.
Our answer: The most important difference between anaerobic and aerobic respiration is the amount of energy captured from each glucose molecule. Aerobic respiration can recharge up to 36 ADP to ATP from each glucose molecule while anaerobic respiration can only recharge 2 ADP.