Transport of Fluids within the Body
14 Cardiovascular and Lymphatic Systems
Introduction to the Cardiovascular and Lymphatic Systems
Humans are complex multicellular organisms that require a mechanism for transporting nutrients throughout their bodies and for removing waste products. The circulatory or cardiovascular system has evolved over time from simple diffusion through cells in the early evolution of animals to a complex network of blood vessels that reach all parts of the human body. This extensive network supplies our cells, tissues, and organs with oxygen and nutrients and removes carbon dioxide and waste, which are byproducts of cellular respiration.
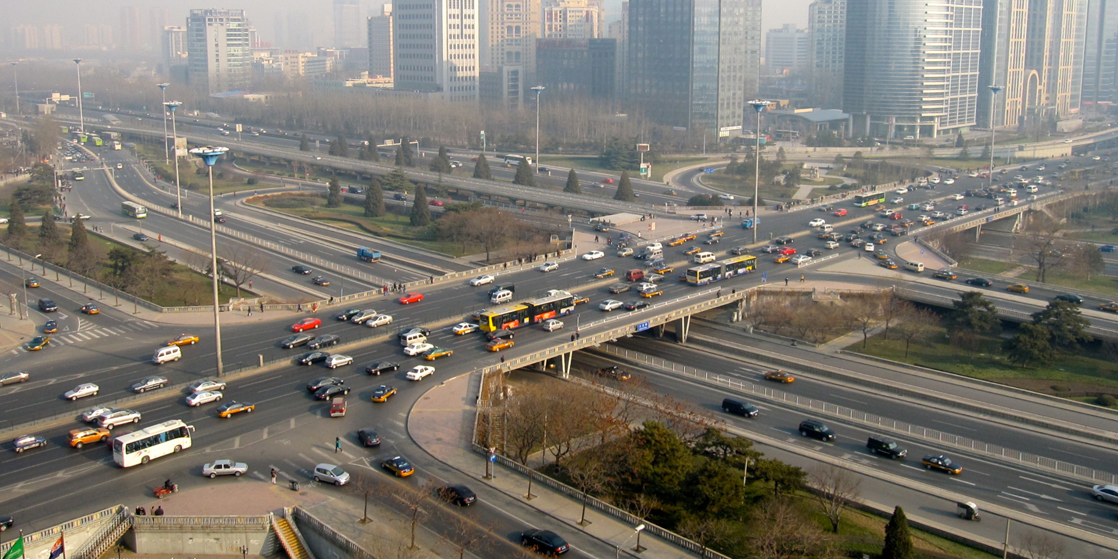
At the core of the human circulatory system is the heart. The size of a clenched fist, the human heart is protected beneath the rib cage. Made of specialized and unique cardiac muscle, it is a two-sided pump that moves blood throughout the body and to the heart itself in a network of blood vessels. Heart contractions are driven by intrinsic electrical impulses that the brain and endocrine hormones help to regulate. Understanding the heart’s basic anatomy and function is important to understanding the body’s circulatory and respiratory systems.
Take any Biology textbook or course, and chances are that the chapter dedicated to the lymphatic system will be short and lacking detail when compared to its mighty and well-known sibling, the cardiovascular system. Maybe because of the prominence and the romantic notions ascribed to the heart and blood, the cardiovascular system has been studied much more extensively. Since its original description by Hippocrates, the lymphatic system has been neglected by both scientific and medical communities because of its vagueness in structure and function. “Rediscovered” in the 1600s as the venae albae et lacteae (“milky veins”), the lymphatic system was for long considered a secondary vascular system that supports the blood vascular system.
In fact, the lymphatic system has important functions ranging from transporting fats and returning leaked fluids to the blood to housing the cells and many organs of the immune response. The immune function of the lymphatic system will be discussed in the next chapter. Refer to the chapter on the digestive system to review the lymphatic system’s role in absorbing lipids during digestion. Here we will focus on its role in returning tissue fluid to the blood.
Circulatory System Structure and Function
The circulatory system is effectively a network of cylindrical vessels: the arteries, veins, and capillaries that begin with a pump, the heart. Blood flow is directed in two circuits: one through the lungs and back to the heart called pulmonary circulation, and the other throughout the rest of the body and its organs including the brain, called systemic circulation. The hearts of animals have four chambers that completely separate the oxygenated and deoxygenated blood. It pumps only oxygenated blood through the body and only deoxygenated blood to the lungs.
The heart is separated from the lungs inside the thorax by a membranous sack called the parietal pericardium. This sack is continuous with the outermost layer of the heart called the visceral epicardium. Just deep to the pericardium is the myocardium, which is the muscle layer of the heart. It comprises 99% of the mass of the heart. The endocardium is the innermost layer of the heart tissue that lines the heart chambers and is continuous with the endothelium lining blood vessels.
With this brief introduction, we will now move to discussions of the molecular, cellular, tissue, and organ levels of organization. Before we turn our attention to the “plumbing” of the cardiovascular system (the heart and blood vessels), we will begin by describing the structure and function of the blood that flows through our bodies.
Levels of Organization in the Cardiovascular System
Molecules and Cells of Blood
Blood is considered fluid connective tissue. This complex tissue is important for the regulation of the body’s systems and homeostasis. Blood helps maintain homeostasis by stabilizing pH, temperature, and osmotic pressure and by eliminating excess heat. Blood supports growth by distributing nutrients and hormones and by removing waste. Blood plays a protective role by transporting clotting factors and platelets to prevent blood loss and transporting the disease-fighting agents or white blood cells to sites of infection.
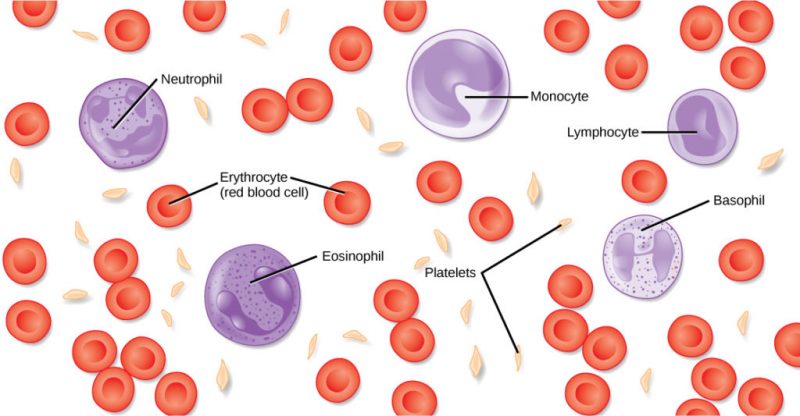
Blood is actually a term used to describe the liquid that moves through the vessels and includes plasma (the liquid portion, which contains water, various proteins, salts, lipids, and glucose), cells (erythrocytes and leukocytes), and cell fragments called platelets. Blood plasma is actually the dominant component of blood and contains proteins, electrolytes, lipids, and glucose in water. The cells are responsible for carrying the gases (erythrocytes) and the immune response (leukocytes). The platelets are responsible for blood clotting. The interstitial fluid that surrounds cells is separate from the blood. In humans, cellular components make up approximately 45 percent of the blood and the liquid plasma 55 percent. Blood is 20 percent of a person’s extracellular fluid and eight percent of weight.
Red Blood Cells
Red blood cells, or erythrocytes (erythro- = “red”; -cyte = “cell”), are specialized cells that circulate through the body delivering oxygen to cells; they are formed from stem cells in the bone marrow. In mammals, red blood cells are small biconcave cells that at maturity do not contain a nucleus or mitochondria and are only 7–8 µm in size. The red coloring of blood comes from the iron-containing protein hemoglobin, shown below. As you have learned, the protein hemoglobin is responsible for distributing oxygen and to a lesser extent, carbon dioxide throughout the circulatory systems of humans.
![]() |
![]() |
Each hemoglobin molecule binds four oxygen molecules so that each red blood cell carries one billion molecules of oxygen. There are approximately 25 trillion red blood cells in the five liters of blood in the human body, which could carry up to 25 sextillion (25 × 1021) molecules of oxygen in the body at any time. In mammals, the lack of organelles in erythrocytes leaves more room for the hemoglobin molecules, and the lack of mitochondria also prevents the use of oxygen for metabolic respiration. Only mammals have anucleated (without a nucleus) red blood cells. Anucleated red blood cells metabolize anaerobically (without oxygen), making use of a primitive metabolic pathway to produce ATP and increase the efficiency of oxygen transport.
The small size and large surface area of red blood cells allow for rapid diffusion of oxygen and carbon dioxide across the plasma membrane. In the lungs, carbon dioxide is released, and oxygen is taken in by the blood. In the tissues, oxygen is released from the blood, and carbon dioxide is bound for transport back to the lungs.
Example: Nitroglycerine
An important characteristic of red blood cells is their glycolipid and glycoprotein coating. These are lipids and proteins that have carbohydrate molecules attached. In humans, the surface glycoproteins and glycolipids on red blood cells vary between individuals, producing the different blood types, such as A, B, and O. Red blood cells have an average life span of 120 days, at which time they are broken down and recycled in the liver and spleen by phagocytic macrophages, a type of white blood cell. Blood Types Related to Proteins on the Surface of the Red Blood Cells
Red blood cells are coated in antigens made of glycolipids and glycoproteins. The composition of these molecules is determined by genetics, which has evolved over time. In humans, the different surface antigens are grouped into 24 different blood groups with more than 100 different antigens on each red blood cell. The two most well-known blood groups are the ABO, shown in the figure above, and the Rh systems. The surface antigens in the ABO blood group are glycolipids, called antigen A and antigen B. People with blood type A have antigen A, those with blood type B have antigen B, those with blood type AB have both antigens, and people with blood type O have neither antigen. Antibodies called agglutinougens are found in the blood plasma and react with the A or B antigens if the two are mixed. When type A and type B blood are combined, agglutination (clumping) of the blood occurs because of antibodies in the plasma that binds with the opposing antigen; this causes clots that coagulate in the kidney causing kidney failure. Type O blood has neither A nor B antigens, and therefore, type O blood can be given to all blood types. Type O negative blood is the universal donor. Type AB-positive blood is the universal acceptor because it has both A and B antigens. The ABO blood groups were discovered in 1900 and 1901 by Karl Landsteiner at the University of Vienna.
The Rh blood group was first discovered in Rhesus monkeys. Most people have the Rh antigen (Rh+) and do not have anti-Rh antibodies in their blood. The few people who do not have the Rh antigen and are Rh– can develop anti-Rh antibodies if exposed to Rh+ blood. This can happen after a blood transfusion or after an Rh– woman has an Rh+ baby. The first exposure does not usually cause a reaction; however, at the second exposure, enough antibodies have built up in the blood to produce a reaction that causes agglutination and the breakdown of red blood cells. An injection can prevent this reaction.
Human red blood cells may have either type A or B glycoproteins on their surface, both glycoproteins combined (AB), or neither (O). The glycoproteins serve as antigens and can elicit an immune response in a person who receives a transfusion containing unfamiliar antigens. Type O blood, which has no A or B antigens, does not elicit an immune response when injected into a person of any blood type. Thus, O is considered the universal donor. Persons with type AB blood can accept blood from any blood type, and type AB is considered the universal acceptor.
White Blood Cells
White blood cells also called leukocytes (leuko = white), are approximately one percent by volume of the cells in the blood. The role of white blood cells is very different than that of red blood cells: they are primarily involved in the immune response to identify and target pathogens, such as invading bacteria, viruses, and other foreign organisms. White blood cells are formed continually; some only live for hours or days, but some live for years.
The morphology of white blood cells differs significantly from red blood cells. They have nuclei and do not contain hemoglobin. The different types of white blood cells are identified by their microscopic appearance after histologic staining, and each has a different specialized function. The two main groups, both illustrated in the figure below, are the granulocytes, which include neutrophils, eosinophils, and basophils, and the agranulocytes, which include monocytes and lymphocytes.
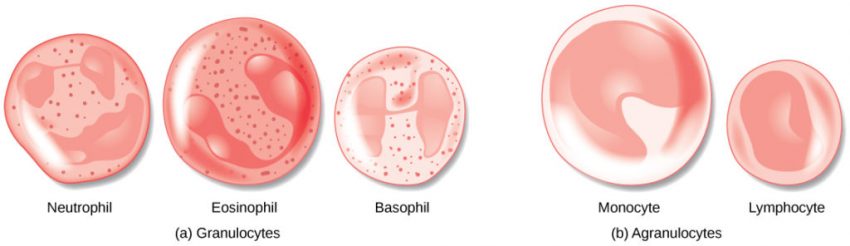
Granulocytes contain granules in their cytoplasm. The agranulocytes are so named because of the lack of granules in their cytoplasm. Some leukocytes become macrophages that either stay at the same site or move through the bloodstream and gather at sites of infection or inflammation where chemical signals from foreign particles and damaged cells attract them. Lymphocytes are the primary cells of the immune system and include B cells, T cells, and natural killer cells. B cells destroy bacteria and inactivate their toxins. They also produce antibodies. T cells attack viruses, fungi, some bacteria, transplanted cells, and cancer cells. T cells attack viruses by releasing toxins that kill the viruses. Natural killer cells attack a variety of infectious microbes and certain tumor cells.
Platelets and Coagulation Factors
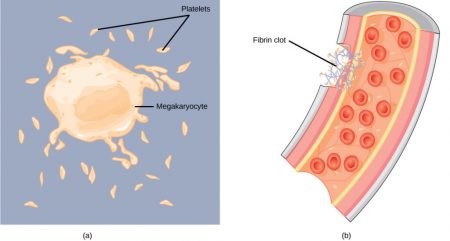
Blood must clot to prevent excess blood loss, a process called hemostasis. Small cell fragments called platelets (thrombocytes) are attracted to the wound site where they adhere by extending many projections and releasing their contents. These contents activate other platelets and also interact with other coagulation factors, which convert fibrinogen, a water-soluble protein present in blood serum into fibrin (a non-water soluble protein), causing the blood to clot. Calcium ions are critical to this conversion.
Many of the clotting factors require Vitamin K to work, and vitamin K deficiency can lead to problems with blood clotting. Many platelets converge and stick together at the wound site forming a platelet plug (also called a fibrin clot). The plug or clot lasts for a number of days and stops the loss of blood. Platelets are formed from the disintegration of larger cells called megakaryocytes. For each megakaryocyte, 2000–3000 platelets are formed with 150,000 to 400,000 platelets present in each cubic millimeter of blood. Each platelet is disc-shaped and 2–4 μm in diameter. They contain many small vesicles but do not contain a nucleus.
The cells of the blood, including all those involved in the immune response, arise in the bone marrow via various differentiation pathways from hematopoietic stem cells. In contrast with embryonic stem cells, hematopoietic(heme = blood, poio = create) stem cells are present throughout adulthood and allow for the continuous differentiation of blood cells to replace those lost to age or function.
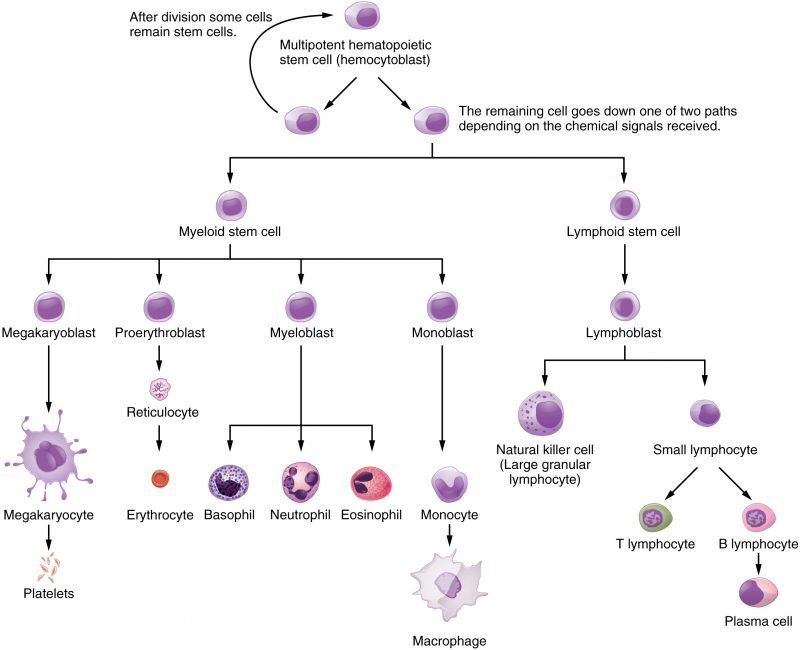
Plasma and Serum
The liquid component of blood is called plasma, and it is separated by spinning or centrifuging the blood at high rotations (3000 rpm or higher). Centrifugal forces separate the blood cells and platelets to the bottom of a specimen tube. The upper liquid layer, the plasma, consists of 90 percent water along with various substances required for maintaining the body’s pH and osmotic load and for protecting the body. The plasma also contains coagulation factors and antibodies.
The plasma component of blood without the coagulation factors is called serum. Serum is similar to interstitial fluid in that the correct composition of key ions acting as electrolytes is essential for the normal functioning of our cells. Other components in the serum include proteins that assist with maintaining pH and osmotic balance while giving viscosity (~thickness) to the blood. The serum also contains antibodies, specialized proteins that are important for defense against viruses and bacteria. Lipids, including cholesterol, are also transported in the serum attached to binding proteins, along with various other substances including nutrients, hormones, metabolic waste, plus external substances, such as drugs, viruses, and bacteria.
Human serum albumin is the most abundant protein in human blood plasma and is synthesized in the liver. Albumins, which constitute about half of the blood serum protein, transport hormones and fatty acids, buffer pH, and maintain osmotic pressures. Immunoglobin is a protein antibody produced in the mucosal lining and plays an important role in antibody-mediated immunity.
Learn By Doing 14.1
Which is the most accurate composition of blood components?
- blood = serum + cells
- blood = plasma + serum
- blood = plasma + serum + clotting factors
- blood = plasma + cells
Which formed element would typically be the smallest?
- platelet
- white blood cell
- red blood cell
Which of the following is not properly matched with its function?
- platelets: hemostasis
- leukocytes: Hemostasis
- white blood cells: Immune function
- red blood cells: carry oxygen
Which type of blood cell contains the greatest amount of iron?
- red blood cells
- granulocyte
- leukocytes
- agranulocytes
Which of the following do not have nuclei?
- red blood cells
- neutrophils (a type of granulocyte)
- platelet
- lymphocytes (a type of agranulocyte)
- red blood cells and platelets
- neutrophils and lymphocytes (both are types of leukocytes)
One of the stages of red blood cell production is called the reticulocyte, so named because these cells contain ribosomal RNA strands that when stained look like reticular fibers. Reticulocytes are those cells recently released by the bone marrow and only circulate for about 24 hours before becoming mature RBCs. Normally about 1% of circulating RBCs are reticulocytes.
In the hours and days after donating blood, what would you expect to happen to both your erythropoietin levels and your reticulocyte count?
- They will both increase.
- Erythropoietin will decrease, and reticulocyte count will increase.
- They will both decrease.
- Erythropoietin will increase, and reticulocyte count will decrease.
Which of the following proteins allows red blood cells to transport oxygen?
- Myoglobin
- Hemoglobin
- Alpha globulin
- Gama globulin
Red blood cells have a limited lifespan because __________.
- they express self-proteins that degrade, causing immune cells to attack them
- their high rate of cell division causes mutations that accumulate over time
- they experience apoptosis (programmed cell death)
- they cannot “fix” damage that accumulates over time as they no longer have DNA
Organs of the Cardiovascular System: Heart and Blood Vessels
Learning Objectives
- Describe the structure of the heart and explain how cardiac muscle is different from other muscles
- Describe the cardiac cycle
- Explain the structure of arteries, veins, and capillaries and how blood flows through the body
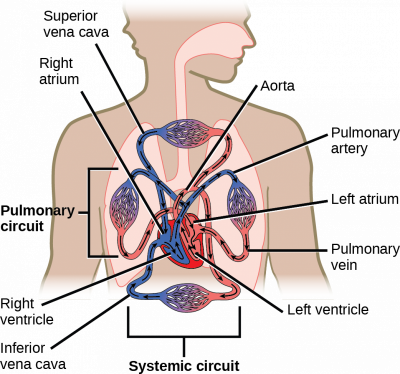
The heart is a complex muscle that pumps blood through the three divisions of the circulatory system: the coronary (vessels that serve the heart), pulmonary (heart and lungs), and systemic (systems of the body), as shown below. Coronary circulation to the heart supplies blood to cardiac muscle and takes blood directly from the main artery (aorta) coming from the heart. For pulmonary and systemic circulation, the heart has to pump blood to the lungs or the rest of the body, respectively. In vertebrates, the lungs are relatively close to the heart in the thoracic cavity. The shorter distance to pump means that the muscle wall on the right side of the heart is not as thick as the left side which must have enough pressure to pump blood all the way to your big toe.
Structure of the Heart
The heart is made of two pumps, each containing an atrium and a ventricle that is separated by a thick wall called a septum. The heart pumps blood in one direction through the circulatory system to provide oxygen to organs and to remove metabolic waste from the tissues. Each heartbeat forces blood through the circulatory system and back to the heart. The blood enters the right atrium and passes into the right ventricle. It is then ejected from the right ventricle into the pulmonary artery, which carries blood from the heart to the lungs. Gas exchange, which involves releasing carbon dioxide and acquiring oxygen, occurs within the capillaries of the lungs. Freshly re-oxygenated blood returns through the pulmonary vein back to the left atrium. The blood passes from the left atrium into the left ventricle. From there, it is ejected into the aorta and then into systemic circulation.
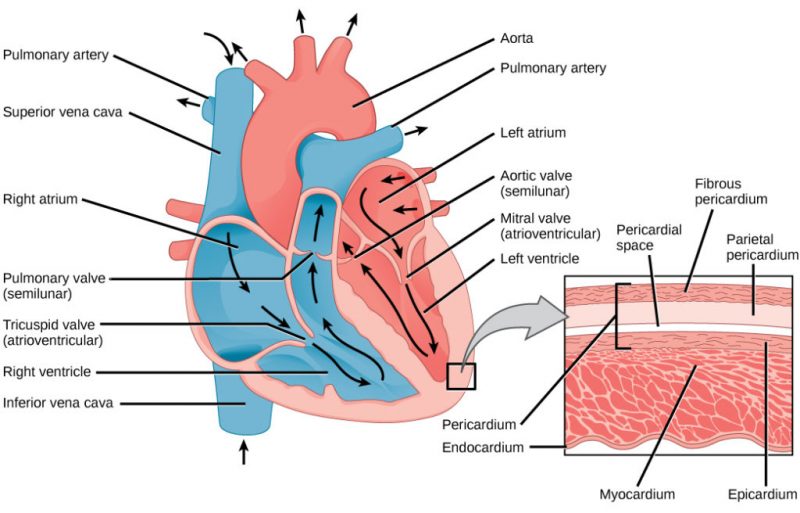
In many ways, the heart is the center of the circulatory system. It is the pump that controls the movement of blood. The arteries carry blood away from the heart; veins carry it back to the heart. Blood circulates around the body, providing nutrients to organs while removing metabolic waste. What route does blood take? Blood enters the right side of the heart by streaming into the right atrium, the chamber of the heart that receives blood from the superior vena cava, inferior vena cava, and coronary sinus.
From the right atrium, it flows into the right ventricle. Blood then exits the heart and enters pulmonary circulation, traveling through the pulmonary artery(carrying blood from the heart to the lungs) to the lungs where gases are exchanged. Freshly re-oxygenated blood returns to the left atrium, traveling through the pulmonary veins(carrying blood from the lungs to the heart). From the left atrium, it flows into the left ventricle before exiting the heart into the aorta to enter the systemic circulation to be transported throughout the body. This oxygen-rich blood flows through the arteries to the organs where oxygen is delivered, and metabolic waste is removed. From the organs, oxygen-poor (more specifically, less oxygenated) blood returns to the heart, carried by the veins, and enters the right atrium again.
While these muscular pumps work together in the heart, they are not equal in the size of their musculature. The left side contains thicker muscles than the right side. Why? The right side has to generate less pressure in order to pump blood through the short circulatory pathway of the lungs. This does not require as much pressure as pumping to the systemic circulation as the left ventricle has to do.
Example: Atrial Septal Defects, Diseases of the Heart
Have you ever heard of having a hole in the heart? A small percentage of people have just that, a hole in the wall (septum) that separates the right and left atria. Medically, this is known as an atrial septal defect. This defect is a congenital disease, meaning it is present at birth. When a fetus is still developing, the septum between the right and the left atrium is not fully formed. The atria are connected together by a hole, called the foramen ovale, between the two chambers. This opening allows blood in the fetal heart to be shunted from the right atrium into the left atrium. This shunted blood does not get pumped into the pulmonary artery, as this is not necessary in the fetus. Instead, the fetus receives oxygen (and nutrients) from the placenta via the umbilical cord. When delivery occurs, and the newborn takes his or her first breath, the lungs inflate. This changes the pressure distribution in the heart and causes a flap of tissue to close the foramen ovale, which then grows into a completed septum. When the foramen ovale does not close properly, or if there are similar congenital defects, the child is left with an atrial septal defect or, in other words, a hole in his or her heart.
Whether or not this problem needs to be corrected is mainly dependent on the size of the hole. This is because some blood from the left atrium will move through the atrial septal defect into the right atrium, causing it to recirculate through pulmonary circulation. If too much blood is shunted back through the lungs, it can cause pulmonary hypertension and ultimately heart failure.
Most of the time, symptoms are very mild or not even noticed. Sometimes, symptoms do not develop until much later in life. Atrial septal defects can be treated with surgical closure of the defect.
Valves of the Heart
As mentioned earlier, blood flows through the heart in one direction. What prevents blood from flowing in the opposite direction? The answer is the heart’s valves. These valves maintain unidirectional flow by preventing backward (retrograde) flow when the pressure gradients change during the heart cycle. These valves are found in matched pairs in two locations of the heart. The first set, the atrioventricular (AV) valves, separate the atria from the ventricles. The second set, the semilunar valves, are located between the ventricles and the arteries they feed.
If we follow blood flow beginning with the right atrium, the first valve is the tricuspid valve that separates the right atrium from the right ventricle. This AV valve is so named because it has three flaps: the anterior cusp, the medial cusp, and the posterior cusp. On the ventricle side of the valve are long muscular cords, called chordae tendinae, that extend from the inferior side of each cusp. The chordae tendinae attach to each cusp on one end and to the papillary muscle, which is connected to the inner wall of the ventricle on the other end. These muscles prevent the valves from inverting back into the atria during ventricular contraction.
Most of the blood flows passively into the atria, through the tricuspid valve, and into the right ventricle. Atrial contractions then push most of the remaining atrial blood into the ventricle. As the right ventricle fills, the pressure inside it increases. This increased pressure, coupled with the right atria moving into its relaxation phase, causes the ventricular pressures to be greater than atrial pressures. This causes the tricuspid AV valves to close. In this closed position, the chordae tendinae that are attached to the ventricular side of the valves are fully extended and taut, like a stretched bungee cord. The chordae tendinae prevent the higher pressure in the ventricle from forcing the valve to open back into the atria, thus preventing the backflow of blood through the tricuspid valve.
As the blood flows from the right ventricle into the pulmonary artery, it passes through the pulmonary semilunar valve (pulmonary valve). Opposite to the tricuspid, the pulmonary semilunar valve remains closed when the ventricle is filling and opens during ventricular contraction. Again, this is because the valves work by opening when the pressure is higher on their proximal side and closing when the pressure is higher on their distal side.
On its return from the lungs, blood enters the left atrium. As the blood passes from the left atrium into the left ventricle, it passes through the bicuspid valve, often referred to as the mitral valve. This valve is similar in structure and function to the tricuspid but only contains two cusps, the anterior and posterior cusps. It, too, is open when the pressure in the atria exceeds the ventricle and it, too, has chordae tendinae and papillary muscles that prevent its inversion upon ventricular contraction. The release of blood from the left ventricle into the aorta is regulated by the aortic semilunar valve, which is most commonly referred to as the aortic valve. It is similar in structure and function to the pulmonary semilunar valve. Because they regulate the flow of blood through the heart and prevent its back-flow, these valves are indispensable for the proper function of the heart.
Blood Flow Through the Heart
Blood circulates in a closed system. It flows from the heart, through the lungs, back to the heart, and then to the rest of the body. The blood always returns to the heart. The amount of blood that circulates and the rate blood circulates is controlled by the pumping of the heart. The system is similar to having a rubber ball filled with water and a tube connected on both sides of it. When the ball is squeezed, the water enters the tube on one side. When the ball is relaxed, water is drawn into it through the other side. Squeeze again, and more water is ejected into the tube. Relax again, and water is drawn into the ball. The valves between the opening of the tube and the ball, which only open one way, control the entry of water into the tube and prevent its tendency to move backward.
Oxygen-poor blood enters the lungs where carbon dioxide, a waste product of our cells, is exchanged for oxygen. The oxygen-rich blood returns to the heart by way of the pulmonary veins. When it arrives from the lungs, it enters the left atrium. Some of the blood flows passively through the bicuspid (or mitral) valve into the left ventricle. When the left atrium contracts, the remaining blood in the left atrium is pushed through the bicuspid valve into the powerful left ventricle.
After the left ventricle contracts, the blood exits through the aortic valve into the aorta to be circulated throughout the body. The first section of the aorta is the ascending aorta, which runs only a short way before becoming the aortic arch at the apex of an inverted U, from which vessels branch to supply the head, neck, and arms. After the aortic arch turns downward, it becomes the descending aorta. The thoracic aorta supplies blood to the thorax above the diaphragm, and the abdominal aorta supplies blood to the region below the diaphragm. Arteries that branch off of the aorta continue to branch into smaller and smaller arterioles and eventually form the capillaries within the organ. It is within the capillaries that the gas exchange occurs. In the organs, oxygen is provided, and carbon dioxide is removed. This is the opposite of gas exchange in the lungs where carbon dioxide is removed, and oxygen is provided.
Once the exchange has occurred, oxygen-poor blood exits the capillaries, flowing into venules or small veins. These venules combine to form veins, which eventually return the blood to the right atrium. The blood from the head, neck, and arms returns to the right atrium through the superior vena cava, while the blood from the lower body returns through the inferior vena cava. The right atrium contracts and blood passes through the tricuspid valve into the right ventricle. From the right ventricle, the blood is ejected through the pulmonary semilunar valve into the pulmonary artery to travel to the lung arterioles and capillaries. Oxygen-rich blood flows into the venules and back to the heart through the pulmonary vein and is ready to enter the left atrium again and circulate around the body again.
The heart is composed of three layers: the epicardium, the myocardium, and the endocardium. The inner wall of the heart has a lining called the endocardium. The myocardium consists of the heart muscle cells that make up the middle layer and the bulk of the heart wall. The outer layer of cells is called the epicardium, of which the second layer is a membranous layered structure called the pericardium that surrounds and protects the heart. The pericardium allows enough room for vigorous pumping but also keeps the heart in place to reduce friction between the heart and other structures.
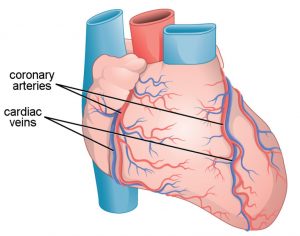
The heart has its own blood vessels that supply the heart muscle with blood. The coronary arteries branch from the aorta and surround the outer surface of the heart like a crown. They diverge into capillaries where the heart muscle is supplied with oxygen before converging again into the coronary veins to take the deoxygenated blood back to the right atrium where the blood will be re-oxygenated through the pulmonary circuit.
Learn By Doing 14.2
Which of the following lists the valves in the order through which the blood flows from the vena cava through the heart?
- tricuspid, pulmonary semilunar, bicuspid, aortic semilunar
- mitral, pulmonary semilunar, bicuspid, aortic semilunar
- aortic semilunar, pulmonary semilunar, tricuspid, bicuspid
- bicuspid, aortic semilunar, tricuspid, pulmonary semilunar
Which chamber initially receives blood from the systemic circuit?
- left atrium
- left ventricle
- right atrium
- right ventricle
Which of the following is not important in preventing back-flow of blood?
- chordae tendineae
- papillary muscles
- AV valves
- endocardium
The myocardium would be the thickest in the ________.
- left atrium
- left ventricle
- right atrium
- right ventricle
The Cardiac Cycle
The main purpose of the heart is to pump blood through the body; it does so in a repeating sequence called the cardiac cycle. The cardiac cycle is the coordination of the filling and emptying of the heart of blood by electrical signals that cause the heart muscles to contract and relax. The human heart beats over 100,000 times per day. In each cardiac cycle, the heart contracts (systole), pushing out the blood and pumping it through the body; this is followed by a relaxation phase (diastole), where the heart fills with blood. The atria contract at the same time, forcing blood through the atrioventricular valves into the ventricles. Closing of the atrioventricular valves produces a monosyllabic “lup” sound. Following a brief delay, the ventricles contract at the same time forcing blood through the semilunar valves into the aorta and the artery transporting blood to the lungs (via the pulmonary artery). Closing of the semilunar valves produces a monosyllabic “dup” sound.
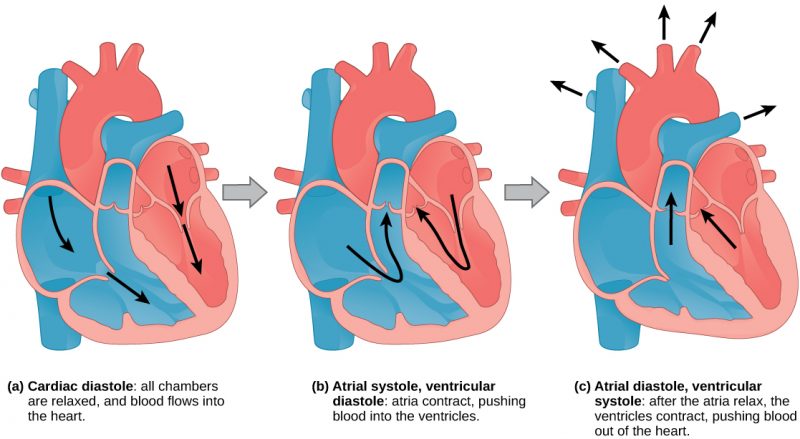
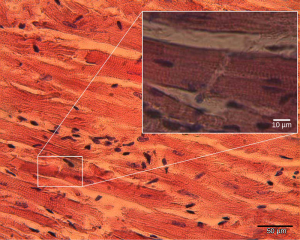
The pumping of the heart is a function of the cardiac muscle cells, or cardiomyocytes, that make up the heart muscle. Cardiomyocytes are distinctive muscle cells that are striated like skeletal muscle but pump rhythmically and involuntarily like smooth muscle; they are connected by intercalated disks exclusive to cardiac muscle. They are self-stimulated for a period of time, and isolated cardiomyocytes will beat if given the correct balance of nutrients and electrolytes.
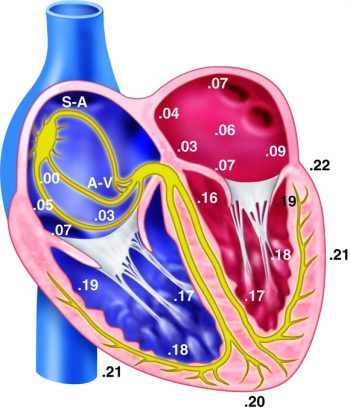
The autonomous beating of cardiac muscle cells is regulated by the heart’s internal pacemaker which uses electrical signals to time the beating of the heart. The electrical signals and mechanical actions are intimately intertwined. The internal pacemaker starts at the sinoatrial (SA) node, which is located near the wall of the right atrium. Electrical charges spontaneously pulse from the SA node causing the two atria to contract in unison. The pulse reaches a second node, called the atrioventricular (AV) node, between the right atrium and right ventricle where it pauses for approximately 0.1 second before spreading to the walls of the ventricles. From the AV node, the electrical impulse enters the bundle of His, then to the left and right bundle branches extending through the interventricular septum. Finally, the Purkinje fibers conduct the impulse from the apex of the heart up the ventricular myocardium, and then the ventricles contract. This pause allows the atria to empty completely into the ventricles before the ventricles pump out the blood. The electrical impulses in the heart produce electrical currents that flow through the body and can be measured on the skin using electrodes. This information can be observed as an electrocardiogram (ECG)—a recording of the electrical impulses of the cardiac muscle.
The beating of the heart is regulated by an electrical impulse that causes the characteristic reading of an ECG. The signal is initiated at the sinoatrial valve. The signal then (a) spreads to the atria, causing them to contract. The signal is (b) delayed at the atrioventricular node before it is passed on to the (c) heart apex. The delay allows the atria to relax before the (d) ventricles contract. The final part of the ECG cycle prepares the heart for the next beat.
Learn By Doing 14.3
Which of the following statements about the circulatory system is false?
- Blood in the pulmonary vein is deoxygenated.
- Blood in the inferior vena cava is deoxygenated.
- Blood in the pulmonary artery is deoxygenated.
- Blood in the aorta is oxygenated.
During the systolic phase of the cardiac cycle, the heart is ________.
- contracting
- relaxing
- contracting and relaxing
- filling with blood
What is the normal pathway for action potential conduction through the heart?
- Bundle of His, AV node, intermodal pathways, SA node, Purkinje fibers
- SA node, intermodal pathways, AV node, Bundle of His, Purkinje fibers
- Purkinje fibers, SA node, intermodal pathways, AV node, Bundle of His
- AV node, intermodal pathways, SA node, Bundle of His, Purkinje fibers
Describe one cardiac cycle, beginning with both atria and ventricles relaxed.
Arteries, Veins, and Capillaries
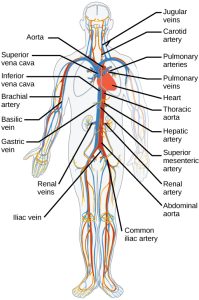
The blood from the heart is carried through the body by a complex network of blood vessels. Arteries take blood away from the heart. The main artery is the aorta which branches into major arteries that take blood to different limbs and organs. These major arteries include the carotid artery that takes blood to the brain, the brachial arteries that take blood to the arms, and the thoracic artery that takes blood to the thorax and then into the hepatic, renal, and gastric arteries for the liver, kidney, and stomach, respectively. The iliac artery takes blood to the lower limbs. The major arteries diverge into minor arteries and then smaller vessels called arterioles to reach more deeply into the muscles and organs of the body.
Arterioles diverge into capillary beds. Capillary beds contain a large number (10 to 100) of capillaries that branch among the cells and tissues of the body. Capillaries are narrow-diameter tubes that can fit red blood cells through in single file and are the sites for the exchange of nutrients, waste, and oxygen with tissues at the cellular level. Fluid also crosses into the interstitial space from the capillaries. The capillaries converge again into venules that connect to minor veins that finally connect to major veins that take blood high in carbon dioxide back to the heart. Veins are blood vessels that bring blood back to the heart. The major veins drain blood from the same organs and limbs that the major arteries supply. Fluid is also brought back to the heart via the lymphatic system.
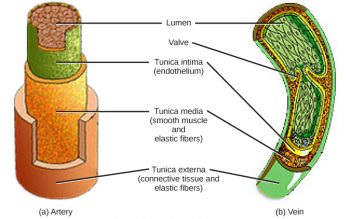
The structure of the different types of blood vessels reflects their function or layers. There are three distinct layers, or tunics, that form the walls of blood vessels. The first tunic is a smooth inner lining of endothelial cells that are in contact with the red blood cells. The endothelial tunic is continuous with the endocardium of the heart. In capillaries, this single layer of cells is the location of diffusion of oxygen and carbon dioxide between the endothelial cells and red blood cells, as well as the exchange site via endocytosis and exocytosis. The movement of materials at the site of capillaries is regulated by vasoconstriction, a narrowing of the blood vessels, and vasodilation, a widening of the blood vessels; this is important in the overall regulation of blood pressure.
Veins and arteries both have two further tunics that surround the endothelium: the middle tunic is composed of smooth muscle, and the outermost layer is connective tissue (collagen and elastic fibers). The elastic connective tissue stretches and supports the blood vessels, and the smooth muscle layer helps regulate blood flow by altering vascular resistance through vasoconstriction and vasodilation. The arteries have thicker smooth muscle and connective tissue than the veins to accommodate the higher pressure and speed of freshly pumped blood. The veins are thinner walled as the pressure and rate of flow are much lower. In addition, veins are structurally different than arteries in that veins have valves to prevent the back-flow of blood. Because veins have to work against gravity to get blood back to the heart, the contraction of skeletal muscle assists with the flow of blood back to the heart.
Learn By Doing 14.4
How do arteries differ from veins?
- Arteries have thicker wall layers to accommodate the changes in pressure from the heart.
- Arteries carry blood.
- Arteries have thinner wall layers and valves and move blood by the action of skeletal muscle.
- Arteries are thin-walled and are used for gas exchange.
How Blood Flows Through the Body
Blood is pushed through the body by the action of the pumping heart. With each rhythmic pump, blood is pushed under high pressure and velocity away from the heart, initially along the main artery, the aorta. In the aorta, the blood travels at 30 cm/sec. As blood moves into the arteries, arterioles, and ultimately to the capillary beds, the rate of movement slows dramatically to about 0.026 cm/sec, one thousand times slower than the rate of movement in the aorta. While the diameter of each individual arteriole and capillary is far narrower than the diameter of the aorta, and according to the law of continuity, fluid should travel faster through a narrower diameter tube, the rate is actually slower due to the overall diameter of all the combined capillaries being far greater than the diameter of the individual aorta.
The slow rate of travel through the capillary beds, which reach almost every cell in the body, assists with gas and nutrient exchange and also promotes the diffusion of fluid into the interstitial space. After the blood has passed through the capillary beds to the venules, veins, and finally to the main venae cavae, the rate of flow increases again but is still much slower than the initial rate in the aorta. Blood primarily moves in the veins by the rhythmic movement of smooth muscle in the vessel wall and by the action of the skeletal muscle as the body moves. Because most veins must move blood against the pull of gravity, blood is prevented from flowing backward in the veins by one-way valves. Because skeletal muscle contraction aids in venous blood flow, it is important to get up and move frequently after long periods of sitting so that blood will not pool in the extremities.
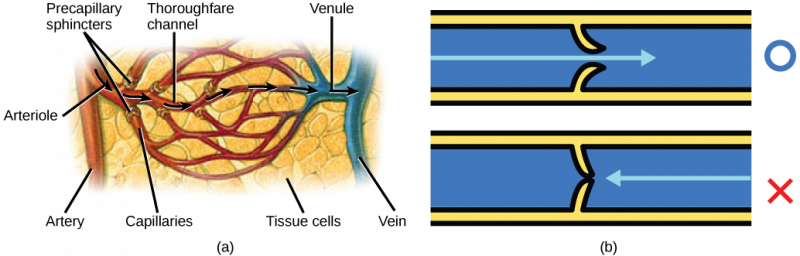
Blood flow through the capillary beds is regulated depending on the body’s needs and is directed by nerve and hormone signals. For example, after a large meal, most of the blood is diverted to the stomach by vasodilation of vessels of the digestive system and vasoconstriction of other vessels. During exercise, blood is diverted to the skeletal muscles through vasodilation while blood to the digestive system would be lessened through vasoconstriction. The blood entering some capillary beds is controlled by small muscles called pre-capillary sphincters. If the sphincters are open, the blood will flow into the associated branches of the capillary blood. If all of the sphincters are closed, then the blood will flow directly from the arteriole to the venule through the thoroughfare channel. These muscles allow the body to precisely control when capillary beds receive blood flow. At any given moment only about 5–10% of our capillary beds actually have blood flowing through them.
Proteins and other large solutes cannot leave the capillaries. The loss of the watery plasma creates a hypertonic solution within the capillaries, especially near the venules. This causes about 85% of the plasma that leaves the capillaries to eventually diffuse back into the capillaries near the venules. The remaining 15% of blood plasma drains out from the interstitial fluid into nearby lymphatic capillaries. We will discuss these further in a later section of this chapter.
Learn By Doing 14.5
Which of the following is the most common path for blood to move from the left ventricle to the right atrium?
- artery, arterioles, capillaries, venules, veins
- capillaries, arterioles, venules, arteries, veins
- veins, venules, capillaries, arterioles, arteries.
- arterioles, arteries, capillaries, venules, veins
What is the color of blood when it is flowing through the systemic veins?
- purple
- blue
- dark red
- bright red
How does the blood in the pulmonary artery compare to blood in the systemic arteries?
Hint: Think about where the pulmonary or systemic arteries are carrying the blood and what will happen there.
Capillary Exchange
Learning Objectives
- Describe the direction of travel for water, glucose, CO2, and oxygen across the capillary.
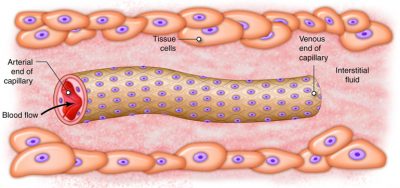
The ultimate goal of the cardiovascular and respiratory systems is to deliver oxygen-rich and nutrient-rich blood to the capillary networks. Although the amount of blood within the capillaries at any given time is only about 5 percent of the total volume, it is the most important blood in the body. It is at the level of the capillaries that capillary exchange occurs. The thin and porous construction of capillary walls provides an avenue for substances such as oxygen and glucose to move out of the blood plasma and into the interstitial fluids surrounding the tissue cells. The reverse process, the uptake of substances such as carbon dioxide and wastes from the interstitial fluids into the plasma, occurs simultaneously. In addition to the back-and-forth exchange of solutes, water also moves between the bloodstream and interstitial fluid in both directions within the capillary networks.
Blood Pressure
Learning Objectives
- Define blood pressure.
- Identify the areas of highest and lowest blood pressure in the cardiovascular system.
Blood pressure (BP)refers to the force per unit area that blood exerts on a vessel wall. It is measured in millimeters of mercury (mmHg). Therefore, the pressure from a column of mercury that is 120 mm high is equivalent to a BP of 120 mmHg. Clinically, such as when your physician checks your BP, it refers to systemic arterial blood pressure in the biggest arteries close to the heart, where BP is similar to that generated by the heart. From these large arteries, blood pressure continuously decreases along each path that blood flow can take through the vascular system. Once the blood gets back to the heart, it is at a very low pressure (near 0 mmHg). The heart then gives it a new “boost” of energy, raising BP again. It is this pressure gradient that continues moving blood as blood moves from areas of higher pressure to areas of lower pressure.
The highest BP is in the aorta and the bigger systemic arteries. In a healthy young adult, BP increases to around 110 mmHg during ventricular systole (contraction) and decreases to around 70 mmHg during ventricular diastole (relaxation). The highest arterial pressure achieved during systole is called systolic blood pressure. The lowest arterial pressure reached during diastole is diastolic blood pressure. The pressure of blood steadily decreases as blood travels farther and farther away from the left ventricle. In the blood that moves from systemic arteries into systemic arterioles and then into capillaries, pressure drops to approximately 35 mmHg. In capillaries, there are no BP fluctuations, but pressure falls to around 16 mmHg when blood reaches the venous end of the capillaries. Because of their distance from the left ventricle, BP in systemic venules and veins drops further. By the time blood reaches the right ventricle, its pressure is typically 0 mmHg. Many factors can affect blood pressure, such as hormones, stress, exercise, eating, sitting, and standing. Blood flow through the body is regulated by the size of blood vessels, by the action of smooth muscle, one-way valves, and the fluid pressure of the blood itself.
Learn By Doing 14.6
What does blood pressure represent?
Hint: Think about the physical push and where it is occurring for your explanation.
Summary of the Cardiovascular System
In humans, the circulatory system is used to transport blood through the body. We use the circulatory system to carry gases, nutrients, and waste through the body. Mammals and birds have a four-chambered heart with no mixing of the blood and double circulation.
Specific components of the blood include red blood cells, white blood cells, platelets, and plasma, which contains coagulation factors and serum. Blood is important for the regulation of the body’s pH, temperature, osmotic pressure, the circulation of nutrients and removal of waste, the distribution of hormones from endocrine glands, and the elimination of excess heat; it also contains components for blood clotting. Red blood cells are specialized cells that contain hemoglobin and circulate through the body delivering oxygen to cells. White blood cells are involved in the immune response to identify and target invading bacteria, viruses, and other foreign organisms; they also recycle waste components, such as old red blood cells. Platelets and blood clotting factors cause the change of the soluble protein fibrinogen to the insoluble protein fibrin at a wound site forming a plug. Plasma consists of 90 percent water along with various substances, such as coagulation factors and antibodies. The serum is the plasma component of the blood without the coagulation factors.
The heart muscle pumps blood through three divisions of the circulatory system: coronary, pulmonary, and systemic. There is one atrium and one ventricle on the right side and one atrium and one ventricle on the left side. The pumping of the heart is a function of cardiomyocytes, distinctive muscle cells that are striated like skeletal muscle but pump rhythmically and involuntarily like a smooth muscle. The internal pacemaker starts at the sinoatrial node, which is located near the wall of the right atrium. Electrical charges pulse from the SA node causing the two atria to contract in unison; then the pulse reaches the atrioventricular node between the right atrium and right ventricle. A pause in the electric signal allows the atria to empty completely into the ventricles before the ventricles pump out the blood. The blood from the heart is carried through the body by a complex network of blood vessels; arteries take blood away from the heart, and veins bring blood back to the heart.
Blood primarily moves through the body by the rhythmic movement of smooth muscle in the vessel wall and by the action of the skeletal muscle as the body moves. Blood is prevented from flowing backward in the veins by one-way valves. Blood flow through the capillary beds is controlled by precapillary sphincters to increase and decrease flow depending on the body’s needs and is directed by nerve and hormone signals. Lymph vessels take fluid that has leaked out of the blood to the lymph nodes where it is cleaned before returning to the heart. During systole, blood enters the arteries, and the artery walls stretch to accommodate the extra blood. During diastole, the artery walls return to normal. The blood pressure of the systole phase and the diastole phase gives the two pressure readings for blood pressure.
Homeostasis and the Cardiovascular System
Homeostatic Imbalances of the Heart
Cardiac output is homeostatically maintained throughout our lifetime so that it constantly meets the needs of the body’s tissues. When the heart becomes damaged, such as after a heart attack, it may not be able to maintain adequate flow. This causes blood pressure to fall, initiating homeostatic feedback loops to try to bring blood pressure (and cardiac output) back to normal.
The heart muscle will die without a steady supply of blood. Atherosclerosis is the blockage of an artery by the buildup of fatty plaques. Because of the size (narrow) of the coronary arteries and their function in serving the heart itself, atherosclerosis can be deadly in these arteries. The slowdown of blood flow and subsequent oxygen deprivation that results from atherosclerosis causes severe pain, known as angina, and complete blockage of the arteries will cause myocardial infarction: the death of cardiac muscle tissue, commonly known as a heart attack. During a heart attack, cells lack oxygen, causing them to die. If enough heart muscle cells die, the heart weakens so that it can’t pump as much blood, leading to the various negative effects associated with this condition.
Congestive heart failure is another common condition affecting cardiac output. It may develop after someone has a heart attack or as a consequence of many other cardiac conditions. The problem with congestive heart failure is that the heart muscle is weakened to the point that it doesn’t do a good job pumping out the blood that is flowing into it (the blood flowing into the heart is called venous return). In fact, one of the main feedback loops that regulate blood pressure does so by adjusting the amount of blood in the body. This is because the more blood we have, the greater our venous return and the higher the cardiac output – assuming a healthy heart. With the weakened heart associated with congestive heart failure, more venous return can actually lead to less cardiac output. A lowered cardiac output decreases blood pressure, signaling the body to increase blood volume, increasing venous return, and making cardiac output lower still. Because this loop continually makes the problem with cardiac output and blood pressure worse, it is a positive feedback loop – and not one we want to experience.
There are mechanisms within the heart that regulate cardiac output. For example, an increased heart muscle contraction will increase with an increase in venous return. Thus, a healthy heart can pump more blood when there is more blood to pump.
There are other controls of heart function and cardiac output from the nervous system and endocrine system, which we will discuss in the integration of systems. It is important to note that we do not consciously change our heart rate or cardiac output. Instead, we can indirectly adjust the nervous and endocrine feedback loops by initiating activities such as exercise or relaxation techniques.
Homeostatic Imbalances of the Plasma Constituents
Although most people realize the importance of red blood cells in distributing oxygen to the body’s tissues, it is important to realize that the plasma (non-cellular) fraction of blood is also critically important. One function of the plasma is to form blood clots when blood vessels are cut or torn in order to prevent excessive blood loss. Blood clotting needs to be limited to the vessels that are damaged in order for the system to function appropriately. Disseminated intravascular coagulation (DIC)is a serious condition in which the clotting cascade becomes activated in many non-injured blood vessels at one time. This occurs secondary to a significant insult to the body such as burns, cancer, infections, etc. With this generalized activation of the clotting cascade, small blood clots are formed, some of which limit or block off the flow of blood within vessels. This cuts off the flow of oxygen to organs and if prolonged, can result in tissue necrosis (cell death), and the organ may stop functioning altogether. The random coagulation throughout the body leads to the coagulation proteins, as well as platelets, being used up, lowering the concentration of the essential clotting factors in the plasma. Once the coagulation process is disrupted, the patient suffers from abnormal bleeding from the GI tract, respiratory tract, and the tiniest wounds in the body.
Homeostatic Imbalances of Red Blood Cell Concentration
Red blood cell concentration is sensitive to changes in oxygen levels. Prolonged exposure to low oxygen causes increased production of RBCs, raising the concentration of red blood cells in the blood (known as hematocrit), in order to maintain homeostatic oxygenation of the tissues. However, hematocrit levels that get too high can also lead to dysfunction, leading to a condition called polycythemia. With polycythemia, the blood will have increased viscosity, making it thicker and harder for the heart to pump this blood through the body. This can lead to heart failure in severe cases.
Homeostatic Imbalances Caused by Vessel Dysfunction
The tissues throughout the body regulate flow to themselves by sending signals that can constrict or dilate the blood vessels that “feed” them. If these vessels don’t have the ability to make the adjustments, the tissues won’t be able to appropriately match blood flow with their metabolic activity.
One example of this is stable angina, where people experience chest pain on exertion. Stable angina is associated with an atherosclerotic plaque that has developed in the main coronary arteries, which deliver flow to the cardiac muscle. Because of the plaque, these vessels can’t dilate appropriately when the needs of the heart muscle increase. Any type of increase in physical activity is not met with normal coronary artery vasodilation in these individuals. This leads to low oxygen levels in their tissues, which can cause pain, as well as prevent the heart from functioning optimally, limiting their activity level.
Autoregulation of Vasoconstrictors and Vasodilators
Autoregulation refers to a tissue’s ability to meet its metabolic needs by automatically altering its blood flow. Autoregulation is particularly important in organs such as the heart and skeletal muscles, whose need for oxygen, nutrients, and the removal of wastes during exercise can be up to 10 times higher than when the body is at rest. In addition to increasing perfusion in the heart and muscles during physical activity, autoregulation manages regional blood flow to other tissues as well, including the brain. Individual mental and physical tasks require significantly different patterns of blood distribution. When a person is on the telephone, for example, motor speech areas of the brain receive more blood when talking, and auditory areas get more blood flow when listening. Autoregulatory changes in blood flow occur in response to local signaling molecules that cause constriction or dilation of local arterioles.
The diameter of blood vessels can be changed by chemicals secreted by various cells, such as white blood cells, smooth muscle cells, platelets, endothelial cells, and macrophages. Metabolically active tissue cells can release vasodilating chemicals such as potassium ions, hydrogen ions, lactic acid, and adenosine. Endothelial cells can also release the vasodilator nitric oxide. Tissue that is injured or inflamed secretes vasodilating compounds of the prostaglandin family. Chemicals that cause vasoconstriction include serotonin (released from platelets) and endothelins (from endothelial cells).
Changes in local oxygen concentrations elicit two very different autoregulatory reactions in the pulmonary and systemic circulations. In systemic circulation, decreased oxygen levels in the tissues cause blood vessels to dilate, increasing the delivery of oxygen. In pulmonary circulation, low oxygen levels in poorly ventilated alveoli cause the blood vessels feeding those alveoli to constrict. As a result, blood avoids most of the alveoli in the lungs that have poor ventilation. Instead, most blood flows to well-ventilated alveoli.
Integration of the Cardiovascular System
Our cardiac output varies with our level of activity, decreasing when resting and increasing when we are more active. For example, cardiac output can increase from the typical 5 L/min at rest up to 15-20 L/min during maximal exercise. When we experience such changes in cardiac output, it is important to realize that not all organs and tissues will experience similar changes in blood flow. So when your cardiac output triples, you will not experience a tripling of flow to each organ. Instead, flow is directed to those tissues that need it most, typically based on their metabolic state. If you are sprinting, your cardiac output might triple, but the flow to the muscles in your legs might go up six or eightfold, while flow to your digestive organs can actually decrease. Thus flow is directed to those capillary beds surrounded by cells with the greatest requirement for oxygen and nutrients.
There are some organs in the body where their level of blood flow is not as dependent on their metabolic need but because they are important in processing and/or interacting with blood in other ways. Examples include the kidneys, which process the blood by removing metabolic wastes, the lungs, which participate in gas exchange; the liver, which processes the substances absorbed in the GI tract, glands, which release hormones into the bloodstream; the skin, which helps regulate body temperature. In the case of the liver and the pituitary gland, they interact with the blood through a portal system, where the vascular network in these tissues contains two capillary beds along the path of blood flow. The kidney blood flow also involves two capillary beds, but these are connected by an arteriole.
The portal system involving the liver plays an important role in processing substances absorbed by the capillaries along the intestines. These capillary beds in the intestines absorb nutrients and other chemicals. This same blood then travels to the liver where it enters the liver sinusoids (a second capillary bed) before returning to the venous circulation. Specific functions occurring in the liver include storing glucose as glycogen, repackaging lipids into lipoproteins, and detoxification of harmful and even useful chemicals, such as drugs. In fact, when discussing the ability of orally administered drugs to distribute throughout the body, the effect of the liver is known as the “first pass” effect.
The kidney actually contains two capillary beds in the same organ, where the first capillary bed (the glomerular capillaries) filters the blood and the second (either the peritubular or vasa recta capillaries) reabsorbs most of the original filtrate.
The cells of the anterior pituitary gland respond to chemical signals secreted into the capillaries of the hypothalamus. Once the blood from the hypothalamus enters the anterior pituitary, it enters a second capillary bed so that the cells of the anterior pituitary can sense these signals and respond by secreting their own hormones if necessary.
Learn By Doing 14.7
Most organs receive blood ____1____ and return blood ____2____.
1 | 2 |
directly from the heart | through other organs back to the heart |
from the heart through another organ | directly to the heart |
directly from the lungs |
Why is the liver an exception to the general rule that blood flows from the heart to a single organ and back to the heart?
- The liver produces hormones that need to travel directly to the pituitary to regulate body functions.
- The liver is responsible for filtering the plasma through its nephrons to remove toxins.
- It is not an exception, because the functions of the liver are so important it only gets blood straight from the heart.
- The liver is responsible for processing substance that were absorbed from the GI tract that may be dangerous to the body before they are transported around the rest of the body.
Neural Regulation of the Cardiovascular System
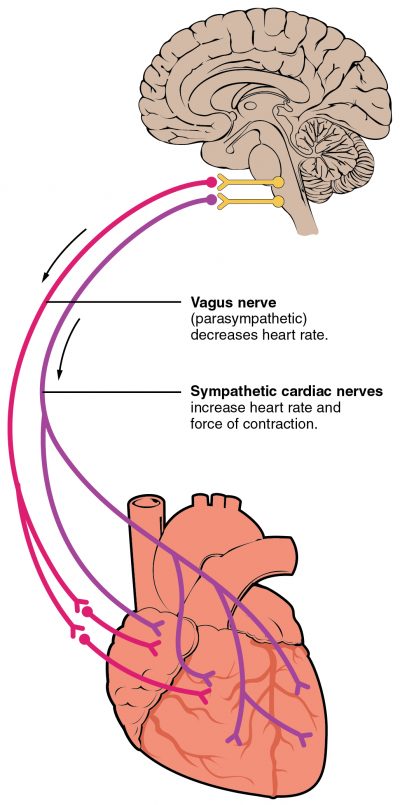
As you have learned, homeostasis relies on communication between body systems, which is primarily carried out by the nervous and endocrine systems. To convey information, the nervous system uses neural impulses, and the endocrine system uses hormones released into the blood. Most homeostatic controls involve negative feedback mechanisms, in which the output of a process either terminates or lessens the original change.
Both the parasympathetic and sympathetic branches of the autonomic nervous system (ANS)alter the cardiac output as necessary. The ANS modulates the heart rate and force of contraction (contractility) of the myocardium.
The parasympathetic nervous system decreases the heart rate. The vagus nerve(the 10th cranial nerve) innervates the SA and AV nodes of the heart. It is the parasympathetic branch of the ANS that sets the heart rate under normal resting conditions. This is commonly referred to as the “vagal tone” as it is the vagus nerve that is responsible for this basal cardiac function. The effect of the parasympathetic system is to decrease the rate at which action potentials are generated at the SA and AV nodes and also to slow down the conduction speed between the SA and the AV nodes.
Conversely, the heart rate is increased through the actions of the sympathetic nervous system. The sympathetic nerves run to the SA node, the AV node, and the myocytes of the ventricles. The sympathetic nerves increase the heart rate by increasing the spontaneous depolarization rate of the SA and AV nodes.
Hormonal Regulation of the Cardiovascular System
A number of hormones are involved in the regulation of blood flow and blood pressure. They work by changing cardiac output, systemic vascular resistance, or total blood volume.
The Renin-Angiotensin-Aldosterone System
The renin-angiotensin-aldosterone system is set in motion when decreases in blood pressure or renal blood flow stimulate cells in the kidney to secrete renin into the blood. This leads to a pathway that ultimately produces the hormone angiotensin II, which increases BP in two ways. First, it causes vasoconstriction. Second, it causes the kidney tubules to reabsorb sodium. High plasma angiotensin is also a trigger for higher levels of aldosterone synthesis by the adrenal cortex. This hormone also acts on the kidneys to increase the reabsorption of sodium ions. The increased reabsorption of Na+ leads to enhanced water reabsorption at the kidneys, and this causes the total blood volume to rise, elevating the BP and leading to an increase in venous return. This increase in the amount of blood flowing into the heart also increases cardiac output.
Epinephrine and Norepinephrine
Sympathetic stimulation promotes the release of epinephrine and norepinephrine by the adrenal medulla. As described above, these hormones (or neurotransmitters) increase the heart rate and force of contraction. This increases cardiac output, which in turn increases arterial pressure. Epinephrine (or norepinephrine) also has the effect of constricting arterioles and veins in the abdominal organs and skin while dilating vessels in the heart and skeletal muscle. This results in increased blood flow to muscles during physical activity.
Antidiuretic Hormone
Decreased blood volume or dehydration prompts an increased release of antidiuretic hormone (ADH) from the posterior pituitary. This hormone, which is produced by the hypothalamus and stored in the posterior pituitary, causes the kidney to retain water. This retention can lead to an increase in blood volume and increasing preload similar to the renin-angiotensin system. At very high levels (such as those released during circulatory shock) it also causes peripheral blood vessels to constrict, helping to raise blood pressure. This is why antidiuretic hormone is also known as vasopressin.
Learn By Doing 14.8
What would happen to heart rate in a person with an epinephrine-secreting tumor? Epinephrine is one of the transmitters of the sympathetic nervous system.
- Heart rate will decrease.
- Heart rate will increase.
Hint: Consider the conditions that typically activate the sympathetic branch of the autonomic nervous system.
Lymphatic System Structure and Function
Learning Objectives
- Describe the 3 major functions of the lymphatic system.
- Identify the origin of lymph.
- Relate imbalances in capillary exchange to edema.
- Compare vascular and lymphatic capillaries.
- Describe the major structures of the lymphatic system.
The lymphatic system, for most people, is associated with the immune system to such a degree that the two systems are virtually indistinguishable. The lymphatic system is the system of vessels, cells, and organs that carries excess fluids to the bloodstream, absorbs and transports dietary lipids, and filters pathogens from the blood. Here we will focus on the basic structure of the lymphatic system and on its role in returning interstitial fluid to the blood. The immune functions of the lymphatic system will be discussed in the next chapter.
A major function of the lymphatic system is to drain body fluids and return them to the bloodstream. Blood pressure causes leakage of fluid from the capillaries, resulting in the accumulation of fluid in the interstitial space—that is, spaces between individual cells in the tissues. In humans, 20 liters of plasma is released into the interstitial space of the tissues each day due to capillary filtration. Once this filtrate is out of the bloodstream and in the tissue spaces, it is referred to as interstitial fluid. Of this, 17 liters is reabsorbed directly by the blood vessels. But what happens to the remaining three liters? This is where the lymphatic system comes into play. It drains the excess fluid and empties it back into the bloodstream via a series of vessels, trunks, and ducts.
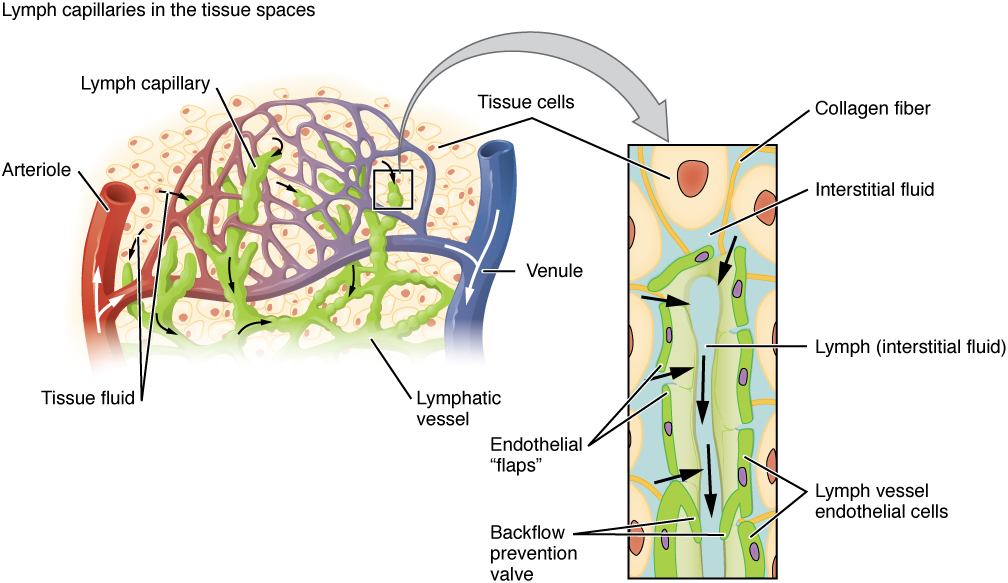
(Credit: Open Stax, CC BY)
Lymphatic capillaries, also called terminal lymphatics, are vessels where interstitial fluid enters the lymphatic system to become lymph. Located in almost every tissue in the body, these vessels are interlaced among the arterioles and venules of the circulatory system in the soft connective tissues of the body. Exceptions are the central nervous system, bone marrow, bones, teeth, and the cornea of the eye, which do not contain lymph vessels.
Lymphatic capillaries are formed by a one-cell-thick layer of endothelial cells and represent the open end of the system, allowing interstitial fluid to flow into them via overlapping cells. When interstitial pressure is low, the endothelial flaps close to prevent “back-flow.” As interstitial pressure increases, the spaces between the cells open up, allowing the fluid to enter. Entry of fluid into lymphatic capillaries is also enabled by the collagen filaments that anchor the capillaries to surrounding structures. As interstitial pressure increases, the filaments pull on the endothelial cell flaps, opening up them even further to allow easy entry of fluid.
A major distinction between the lymphatic and cardiovascular systems in humans is that lymph is not actively pumped by the heart but is forced through the vessels by the movements of the body, the contraction of skeletal muscles during body movements, and breathing. One-way valves (semi-lunar valves) in lymphatic vessels keep the lymph moving toward the heart. Lymph flows from the lymphatic capillaries through lymphatic vessels and then is dumped into the circulatory system via the lymphatic ducts located at the junction of the jugular and subclavian veins in the neck.
Lymph is the term used to describe interstitial fluid once it has entered the lymphatic system. (Lymph becomes white after passing through the lymph nodes and other lymphatic organs due to the infusion of white blood cells.)Lymph moves about the body through the lymphatic system, which is made up of vessels, lymph ducts, lymph glands, and organs, such as tonsils, adenoids, thymus, and spleen
![]() |
![]() (Credit: Medical doctors, CC BY-SA 4.0) |
When the lymphatic system is damaged in some way, such as by being blocked by cancer cells or destroyed by injury, protein-rich interstitial fluid accumulates (sometimes “backs up” from the lymph vessels) in the tissue spaces. This inappropriate accumulation of fluid referred to as lymphedema may lead to serious medical consequences.
Learn By Doing 14.9
To which choice is lymph most similar?
- plasma
- serum
- interstitial fluid
- intracellular fluid
- blood
Blockage of the lymph system can result in which of the following?
- Swelling or edema in the tissues in the area of the blockage
- A reduction in the amount of protein returned to the bloodstream
- Both of the above
Structures of the Lymphatic System
The primary lymphoid organs are the bone marrow and thymus gland. The primary lymphoid organs are where white blood cells called lymphocytes mature, proliferate, and are selected, which enables them to attack pathogens without harming the cells of the body. The secondary lymphatic organs are the locations where lymphocytes act against pathogens. These include the lymph nodes, lymphatic nodules, and spleen.
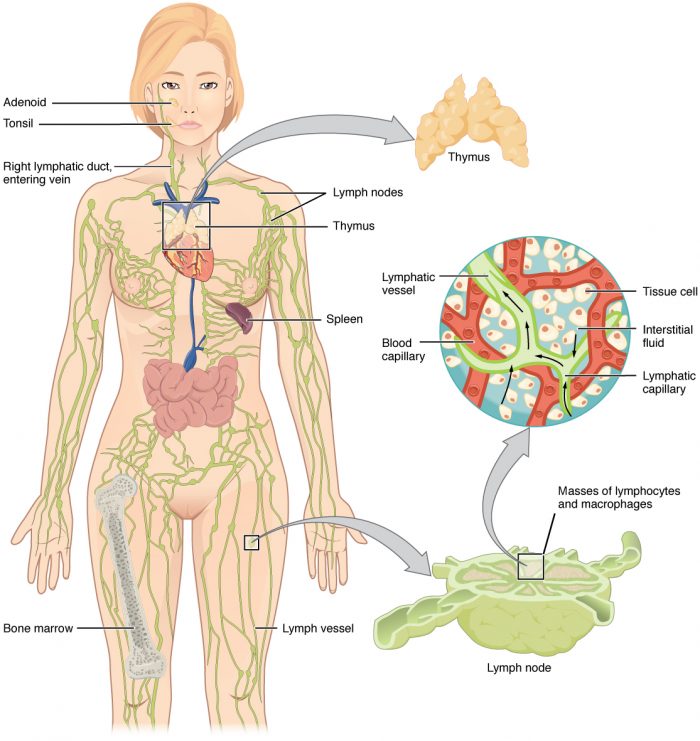
Primary Lymphatic Organs
Bone Marrow
In the embryo, blood cells are made in the yolk sac. As development proceeds, this function is taken over by the spleen, lymph nodes, and liver. Later, the bone marrow takes over most hematopoietic functions, although the final stages of the differentiation of some cells may take place in other organs. The redbone marrow is a loose collection of cells where hematopoiesis occurs. B lymphocytes mature and undergo selection in the bone marrow.
Thymus
The thymus gland is a bilobed organ found in the space between the sternum and the aorta of the heart. Connective tissue holds the lobes closely together but also separates them and forms a capsule.T lymphocytes mature and undergo selection in the thymus. This organ also has an endocrine role, which will be briefly discussed in a later chapter.
The thymus enlarges during childhood and atrophies at puberty. Unlike many other organs, the thymus is at its largest in childhood. The thymus reaches maximum weight (20 to 37 grams) by the time of puberty. The thymus of older people is scarcely distinguishable from surrounding fatty tissue. With aging the thymus slowly shrinks, eventually degenerating into tiny islands of fatty tissue. By the age of 75 years, the thymus weighs only 6 grams. In children, the thymus is grayish-pink in color, and in adults it is yellow.
Secondary Lymphatic Organs
Lymph Nodes
A lymph node is one of the small, bean-shaped organs located throughout the lymphatic system. Any bacteria that infect the interstitial fluid are taken up by the lymphatic capillaries and transported to a regional lymph node, entering through multiple afferent vessels. Macrophages within this organ internalize and kill many of the pathogens that pass through, thereby removing them from the body. The lymph node is also the site of adaptive immune responses mediated by cells of the adaptive immune system. Like the thymus, the bean-shaped lymph nodes are surrounded by a tough capsule of connective tissue and are separated into compartments.
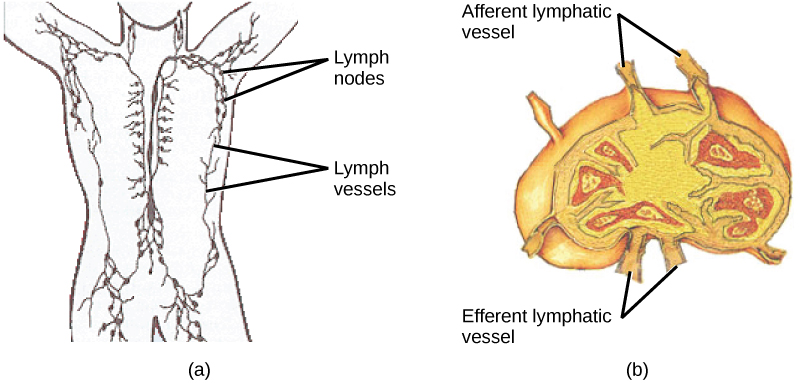
Spleen
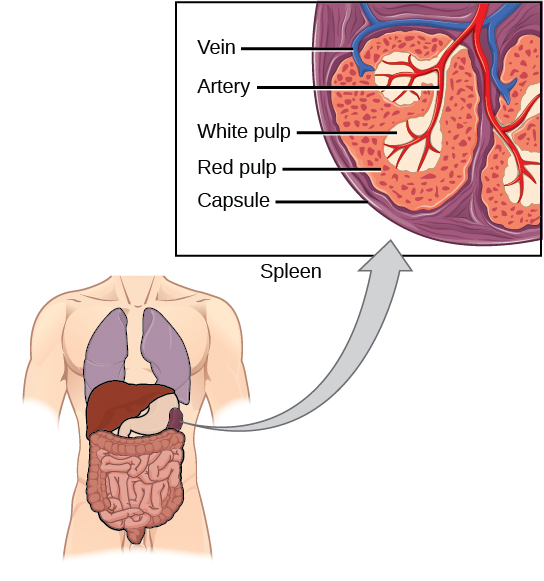
In addition to the lymph nodes, the spleen is a major secondary lymphoid organ. Located in the upper abdomen, it filters both pathogens and worn-out erythrocytes from the blood, and then lymphocytes in the spleen destroy them. The spleen is similar to a lymph node but is much larger and filters blood instead of lymph. The spleen is also divided by bridges of connective tissue, and within each nodule is an area of red pulp, consisting of mostly red blood cells, and white pulp, whose tissue is similar to that inside a lymph node. Blood enters the spleen through arteries and exits through veins.
Lymphoid Nodules
The other lymphoid tissues, thelymphoid nodules, have a simpler architecture than the spleen and lymph nodes in that they consist of a dense cluster of lymphocytes without a surrounding fibrous capsule. These nodules are located in the respiratory and digestive tracts, areas routinely exposed to environmental pathogens. Tonsils are lymphoid nodules located along the inner surface of the pharynx and are important in developing immunity to oral pathogens. The Peyer’s patches of the small intestine are another example of lymphatic nodules.
Example: pharyngeal tonsil
Larger Lymphatic Vessels, Trunks, and Ducts
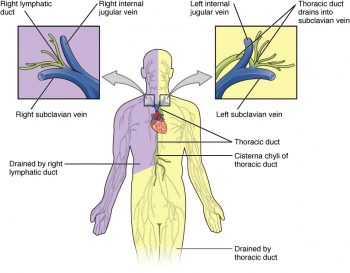
As you’ve read, the lymphatic vessels begin as open-ended capillaries, which feed into larger and larger lymphatic vessels and eventually empty into the bloodstream by a series of ducts. Along the way, the lymph travels through the lymph nodes, which are commonly found near the groin, armpits, neck, chest, and abdomen. Humans have about 500–600 lymph nodes throughout the body.
The lymphatic capillaries empty into larger lymphatic vessels, which are similar to veins. These one-way valves are located fairly close to one another, and each one causes a bulge in the lymphatic vessel, giving the vessels a beaded appearance. The superficial and deep lymphatics eventually merge to form larger lymphatic vessels known as lymphatic trunks. On the right side of the body, the right sides of the head, thorax, and right upper limb drain lymph fluid into the right subclavian vein via the right lymphatic duct. On the left side of the body, the remaining portions of the body drain into the larger thoracic duct, which drains into the left subclavian vein. The thoracic duct itself begins just beneath the diaphragm in the cisterna chyli, a sac-like chamber that receives lymph from the lower abdomen, pelvis, and lower limbs by way of the left and right lumbar trunks and the intestinal trunk.
The overall drainage system of the body is asymmetrical. The right lymphatic duct receives lymph from only the upper right side of the body. The lymph from the rest of the body enters the bloodstream through the thoracic duct via all the remaining lymphatic trunks. In general, lymphatic vessels of the subcutaneous tissues of the skin, that is, the superficial lymphatics, follow the same routes as veins, whereas the deep lymphatic vessels of the viscera generally follow the paths of arteries.
Summary of the Lymphatic System
The lymphatic system is a series of vessels, ducts, and trunks that remove interstitial fluid from the tissues and return it to the blood. The lymphatic vessels are also used to transport dietary lipids and cells of the immune system. Cells of the immune system all come from the hematopoietic system of the bone marrow. The primary lymphoid organs, bone marrow and thymus gland, are the locations where lymphocytes of the adaptive immune system proliferate and mature. Secondary lymphoid organs are sites in which mature lymphocytes congregate to mount immune responses. Many immune system cells use the lymphatic and circulatory systems for transport throughout the body to search for and then protect against pathogens.
Learn By Doing 14.10
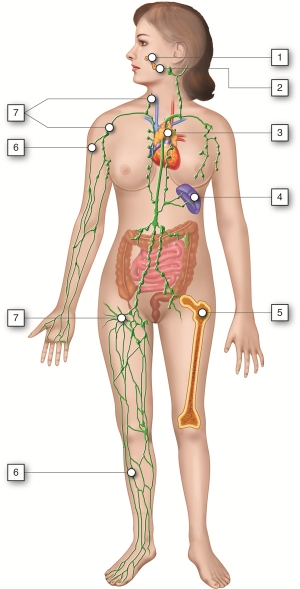
Identify the lymphatic organs in the image. Choose from the following:
- Lymphatic Vessels
- Lymph Nodes
- Pharyngeal Tonsils (adenoids)
- Red Bone Marrow
- Spleen
- Thymus
- Tonsils
What lymphatic organ is inferior to the thymus?
Hint: The organ you are asked to identify is the largest organ in the lymphatic and immune systems.
- Spleen
- Lymph nodes
- Tonsils
- The thymus is the most inferior lymph organ.
Select the correct statement about lymph transport.
- Under normal conditions, lymph vessels are very high-pressure conduits.
- Lymph transport depends on the movement of adjacent tissues, such as skeletal muscles.
- Lymph transport is faster than that occurring in veins.
- Lymph transport is only necessary when illness causes tissue swelling.
Which of the following is NOT a difference between lymphatic and blood circulation?
- Lymphatic capillaries are more permeable than blood capillaries.
- There is no pump in the lymphatic circulation.
- Lymphatic circulation is one-way.
- Skeletal muscle contraction helps flow in lymphatic circulation.
Vascular capillaries tend to leak. Consequently, the fluid that has leaked out of them needs to be drained away so that it does not accumulate in the tissues. How is this accomplished?
- If there is an infection the lymph system picks things up, but otherwise, the blood vessels do.
- The fluid does accumulate, which is why your feet swell on an airplane.
- The fluid, which is very much like serum, is collected through the blind end if the lymph capillary, then filtered through lymph nodes on the way to being returned to the cardiovascular system.
- The fluid leaks back into the capillaries and the proteins end up in the lymph.
Finish the paragraph below by replacing each number with a choice from the matching numbered column.
When thinking about the way the lymph system handles the interstitial fluid, it may be helpful to visualize an area after very heavy rain. The ground becomes saturated, so water and some minerals leach out of the ground and collect in puddles. This would be analogous to___1___ accumulating outside of the circulatory system and in the tissues. When this happens, we often refer to the area with accumulated fluid as being ___2___. Eventually, this excess fluid finds its way to the ___3___, which are pretty leaky and don’t really filter the fluid, much the same way a small stream will accumulate the run-off water after the rain. The fluid inside the lymph capillary is now called ___4___and is pale yellow in color. The lymph fluid is propelled through the capillary which drains into larger lymph ___5___ much the same way a small stream empties into a larger creek. The larger creek usually empties into a river. In the case of this analogy, the larger lymph ___6___ run parallel to the blood vessel. The lymph in the large vessels drains into the larger ___7___ near the heart in the chest. Like a river with a beaver dam, along the way the lymph vessels drain into ___8___which removes some of the debris and foreign matter found circulating in the lymph, slowing down the fluid flow and allowing the cells of the immune system to do their job.
1 |
2 |
3 |
4 |
5, 6 |
7 |
8 |
blood | overhydrated | arterioles | interstitial fluid | nodes | venous vessels | kidney nephrons |
serum | edematous | kidney tubules | lymph | spleen | aorta | lymph nodes |
water | squishy | lymphatic capillaries | water | vessels | ducts | blood capillaries |
interstitial fluid | underhydrated | ducts | arteries |
Homeostasis and the Lymphatic System
The proper functioning of body cells depends on precise regulation of the composition of the interstitial fluid surrounding them. The composition of interstitial fluid changes as substances moves back and forth between it and blood and lymph. In addition, some disruptions come from the external environment.
Fortunately, the body has mechanisms to try to inhibit foreign substances from entering the internal environment of the body, and if they do get in, immune responses help to remove or neutralize the invader before the disruption leads to disease. Unfortunately, some factors or situations affecting the lymphatic system and immune responses could disrupt homeostasis. Since we have already discussed lymphedema, and because the functions of the lymphatic and immune systems are so intertwined, we will discuss failures of homeostasis for the lymphatic system in the chapter on the immune system.
Integration of the Lymphatic System
Learning Objective
All of the systems of the human body functioning together comprise the total human organism, the highest level of organization. All body systems influence and interact with one another. They work together to maintain health and provide protection from disease. The lymphatic system returns filtered fluids to blood preventing plasma loss and interstitial edema. Water and solute must be correctly proportioned in the various compartments of the body to maintain fluid balance. Fluid balance allows for healthy circulation of substances throughout the body, optimal distances for the diffusion of ions and molecules, and proper ion concentration for excitable tissues. In addition, the lymphatic system helps protect the whole organism from the external invasion of foreign substances into the body that may cause disease and also removes damaged or worn-out body cells as a normal component of tissue repair and maintenance.
The following table highlights some of the lymphatic system’s interaction with several other body systems.
Body System | Lymphatic System Support | Lymphatic System Benefits/Effects |
Cardiovascular | Returns filtered fluids and proteins to blood preventing interstitial edema and loss of plasma volume. | Provides transport for white blood cells from site of their origin to their effector site. |
Muscular | Fluid balance provides proper ion concentration and subsequent membrane potentials for contraction | Skeletal muscle pumps help lymph flow through lymphatic vessels |
Respiratory | Prevents interstitial fluid accumulation, so that distance for gas exchange is optimal | Gas exchanges in the lungs provide oxygen to lymphatic cells and remove waste carbon dioxide from these cells necessary for the viability of cells |
Urinary | Assists kidneys in fluid balance by helping maintain fluid in the vascular compartments | Urine flow helps prevent pathogen entrance into the body as part of innate immunity |
Digestive | Lymphatic lacteals transport dietary lipids from the small intestine to the blood | Provides nutrients for lymphoid organs |
Learn By Doing 14.11
The lymphatic system is unique because it serves the body in two very important ways. How? Select the best answer.
- The lymphatic system drains fats from the intestinal tract, and it can sometimes cause autoimmune diseases.
- The lymphatic system helps the body by fighting against infectious agents and looking for abnormal cells that may cause cancer.
- The lymphatic system helps clean up cellular debris and dead bacteria while defining your own personal tissue type.
- The lymphatic system both provides immunity and helps the tissues to keep proper fluid and molecular balance.
The lymphatic system contributes to the body’s homeostasis by performing which of the following functions?
- Draining fluids away from the tissues and returning them to the blood
- Helping to move large molecules around the body
- Providing defense against invading foreign organisms
- All are correct
*
“Learn By Doing” and “Did I Get This?” Feedback
Learn By Doing 14.1
Which is the most accurate composition of blood components?
- blood = serum + cells
- blood = plasma + serum
- blood = plasma + serum + clotting factors
- blood = plasma + cells
Which formed element would typically be the smallest?
- platelet
- white blood cell
- red blood cell
Which of the following is not properly matched with its function?
- platelets: hemostasis
- leukocytes: Hemostasis
- white blood cells: Immune function
- red blood cells: carry oxygen
Which type of blood cell contains the greatest amount of iron?
- red blood cells
- granulocyte
- leukocytes
- agranulocytes
Which of the following do not have nuclei?
- red blood cells
- neutrophils (a type of granulocyte)
- platelet
- lymphocytes (a type of agranulocyte)
- red blood cells and platelets
- neutrophils and lymphocytes (both are types of leukocytes)
One of the stages of red blood cell production is called the reticulocyte, so named because these cells contain ribosomal RNA strands that when stained look like reticular fibers. Reticulocytes are those cells recently released by the bone marrow and only circulate for about 24 hours before becoming mature RBCs. Normally about 1% of circulating RBCs are reticulocytes.
In the hours and days after donating blood, what would you expect to happen to both your erythropoietin levels and your reticulocyte count?
- They will both increase.
- Erythropoeitin will decrease and reticulocyte count will increase.
- They will both decrease.
- Erythropoeitin will increase and reticulocyte count will decrease.
Which of the following proteins allows red blood cells to transport oxygen?
- Myoglobin
- Hemoglobin
- Alpha globulin
- Gama globulin
Red blood cells have a limited lifespan because __________.
- they express self-proteins that degrade, causing immune cells to attack them
- their high rate of cell division causes mutations that accumulate over time
- they experience apoptosis (programmed cell death)
- they cannot “fix” damage that accumulates over time as they no longer have DNA
Learn By Doing 14.2
Which of the following lists the valves in the order through which the blood flows from the vena cava through the heart?
- tricuspid, pulmonary semilunar, bicuspid, aortic semilunar
- mitral, pulmonary semilunar, bicuspid, aortic semilunar
- aortic semilunar, pulmonary semilunar, tricuspid, bicuspid
- bicuspid, aortic semilunar, tricuspid, pulmonary semilunar
Which chamber initially receives blood from the systemic circuit?
- left atrium
- left ventricle
- right atrium
- right ventricle
Which of the following is not important in preventing back-flow of blood?
- chordae tendineae
- papillary muscles
- AV valves
- endocardium
The myocardium would be the thickest in the ________.
- left atrium
- left ventricle
- right atrium
- right ventricle
Learn By Doing 14.3
Which of the following statements about the circulatory system is false?
- Blood in the pulmonary vein is deoxygenated.
- Blood in the inferior vena cava is deoxygenated.
- Blood in the pulmonary artery is deoxygenated.
- Blood in the aorta is oxygenated.
During the systolic phase of the cardiac cycle, the heart is ________.
- contracting
- relaxing
- contracting and relaxing
- filling with blood
Describe one cardiac cycle, beginning with both atria and ventricles relaxed.
Our Answer: The cardiac cycle comprises a complete relaxation and contraction of both the atria and ventricles and lasts approximately 0.8 seconds. Beginning with all chambers in diastole, blood flows passively from the veins into the atria and past the atrioventricular valves into the ventricles. The atria begin to contract following depolarization of the atria and pump blood into the ventricles. The ventricles begin to contract, raising the pressure within the ventricles. When ventricular pressure rises above the pressure in the two major arteries, blood pushes open the two semilunar valves and moves into the pulmonary trunk and aorta in the ventricular ejection phase. Following ventricular repolarization, the ventricles begin to relax, and pressure within the ventricles drops. When the pressure falls below that of the atria, blood moves from the atria into the ventricles, opening the atrioventricular valves and marking one complete heart cycle.
Learn By Doing 14.4
How do arteries differ from veins?
- Arteries have thicker wall layers to accommodate the changes in pressure from the heart.
- Arteries carry blood.
- Arteries have thinner wall layers and valves and move blood by the action of skeletal muscle.
- Arteries are thin-walled and are used for gas exchange.
Learn By Doing 14.5
Which of the following is the most common path for blood to move from the left ventricle to the right atrium?
- artery, arterioles, capillaries, venules, veins
- capillaries, arterioles, venules, arteries, veins
- veins, venules, capillaries, arterioles, arteries.
- arterioles, arteries, capillaries, venules, veins
What is the color of blood when it is flowing through the systemic veins?
- purple
- blue
- dark red
- bright red
How does the blood in the pulmonary artery compare to blood in the systemic arteries?
Our Answer: Blood in the pulmonary artery is less oxygenated than the blood in the systemic arteries. This is reversed in the venous vessels, where the pulmonary veins carry blood that is more oxygenated than the systemic veins.
Learn By Doing 14.6
Our Answer: The force that the blood exerts on the walls of the vasculature or the walls of the chambers of the heart.
Learn By Doing 14.7
Most organs receive blood ____directly from the heart____ and return blood ____directly from the heart____.
1 | 2 |
directly from the heart | through other organs back to the heart |
from the heart through another organ | directly to the heart |
directly from the lungs |
Why is the liver an exception to the general rule that blood flows from the heart to a single organ and back to the heart?
- The liver produces hormones that need to travel directly to the pituitary to regulate body functions.
- The liver is responsible for filtering the plasma through its nephrons to remove toxins.
- It is not an exception because the functions of the liver are so important it only gets blood straight from the heart.
- The liver is responsible for processing substances that were absorbed from the GI tract that may be dangerous to the body before they are transported around the rest of the body.
Learn By Doing 14.8
What would happen to the heart rate in a person with an epinephrine-secreting tumor? Epinephrine is one of the transmitters of the sympathetic nervous system.
- Heart rate will decrease.
- Heart rate will increase.
Learn By Doing 14.9
To which choice is lymph most similar?
- plasma
- serum
- interstitial fluid(Indeed, interstitial fluid is the same as lymph, aside from where it’s found. The latter is inside the lymphatic vessels.)
- intracellular fluid
- blood
Blockage of the lymph system can result in which of the following?
- Swelling or edema in the tissues in the area of the blockage
- A reduction in the amount of protein returned to the bloodstream
- Both of the above
Learn By Doing 14.10
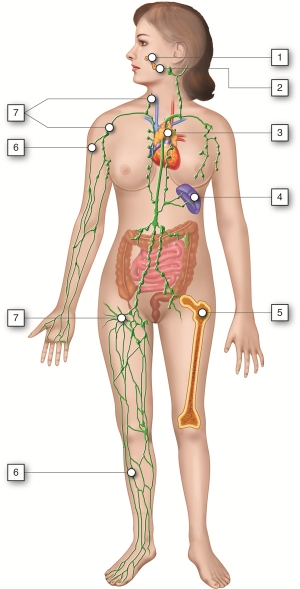
Identify the lymphatic organs in the image. Choose from the following:
- Lymphatic Vessels6,
- Lymph Nodes7
- Pharyngeal Tonsils (adenoids)1
- Red Bone Marrow5
- Spleen4
- Thymus3
- Tonsils2
What lymphatic organ is inferior to the thymus?
Hint: The organ you are asked to identify is the largest organ in the lymphatic and immune systems.
- Spleen
- Lymph nodes
- Tonsils
- The thymus is the most inferior lymph organ.
Select the correct statement about lymph transport.
- Under normal conditions, lymph vessels are very high-pressure conduits.
- Lymph transport depends on the movement of adjacent tissues, such as skeletal muscles.
- Lymph transport is faster than that occurring in veins.
- Lymph transport is only necessary when illness causes tissue swelling.
Which of the following is NOT a difference between lymphatic and blood circulation?
- Lymphatic capillaries are more permeable than blood capillaries.
- There is no pump in the lymphatic circulation.
- Lymphatic circulation is one-way.
- Skeletal muscle contraction helps flow in lymphatic circulation. (In both, contraction of skeletal muscles helps the flow of blood/lymph.)
Vascular capillaries tend to leak. Consequently, the fluid that has leaked out of them needs to be drained away so that it does not accumulate in the tissues. How is this accomplished?
- If there is an infection the lymph system picks things up, but otherwise, the blood vessels do.
- The fluid does accumulate, which is why your feet swell on an airplane.
- The fluid, which is very much like the serum, is collected through the blind end of the lymph capillary, then filtered through lymph nodes on the way to being returned to the cardiovascular system. (The lymph vessels pick up everything except the red and white blood cells.)
- The fluid leaks back into the capillaries and the proteins end up in the lymph.
Finish the paragraph below by replacing each number with a choice from the matching numbered column.
When thinking about the way the lymph system handles the interstitial fluid, it may be helpful to visualize an area after very heavy rain. The ground becomes saturated, so water and some minerals leach out of the ground and collect in puddles. This would be analogous to __interstitial fluid__ accumulating outside of the circulatory system and in the tissues. When this happens, we often refer to the area with accumulated fluid as being ___edematous___. Eventually, this excess fluid finds its way to the ___lymphatic capillaries___, which are pretty leaky and don’t really filter the fluid, much the same way a small stream will accumulate the run-off water after the rain. The fluid inside the lymph capillary is now called ___lymph___ and is pale yellow in color. The lymph fluid is propelled through the capillary which drains into larger lymph ___vessels___ much the same way a small stream empties into a larger creek. The larger creek usually empties into a river. In the case of this analogy, the larger lymph ___ducts___ run parallel to the blood vessel. The lymph in the large vessels drains into the larger ___venous vessels___ near the heart in the chest. Like a river with a beaver dam, along the way the lymph vessels drain into ___lymph nodes___which remove some of the debris, and foreign matter found circulating in the lymph, slowing down the fluid flow and allowing the cells of the immune system to do their job.
1 |
2 |
3 |
4 |
5, 6 |
7 |
8 |
blood | overhydrated | arterioles | interstitial fluid | nodes | venous vessels | kidney nephrons |
serum | edematous | kidney tubules | lymph | spleen | aorta | lymph nodes |
water | squishy | lymphatic capillaries | water | vessels | ducts | blood capillaries |
interstitial fluid | underhydrated | ducts | arteries |
Learn By Doing 14.11
The lymphatic system is unique because it serves the body in two very important ways. How? Select the best answer.
- The lymphatic system drains fats from the intestinal tract, and it can sometimes cause autoimmune diseases.
- The lymphatic system helps the body by fighting against infectious agents and looking for abnormal cells that may cause cancer.
- The lymphatic system helps clean up cellular debris and dead bacteria while defining your own personal tissue type.
- The lymphatic system both provides immunity and helps the tissues to keep proper fluid and molecular balance.(This answer describes the big picture of lymphatic function.)
The lymphatic system contributes to the body’s homeostasis by performing which of the following functions?
- Draining fluids away from the tissues and returning them to the blood
Not quite. While this function is essential, it doesn’t take immunity into account. - Helping to move large molecules around the body
Not quite. This is very important for tissue homeostasis but ignores the rest of the body. - Providing defense against invading foreign organisms
Not quite. This is only one of the important contributions the lymphatic system makes to homeostasis. - All are correct(Correct. These are all important functions.)