Levels of Organization
4 Chemistry
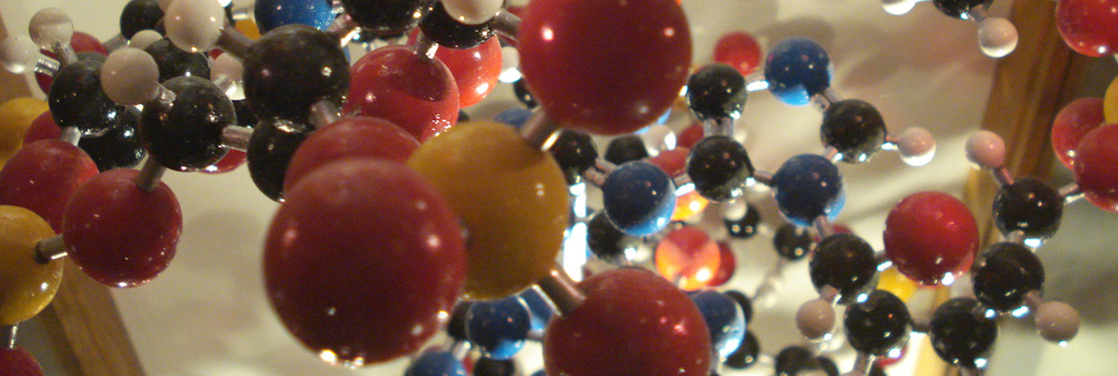
Take a few elements– carbon, hydrogen, nitrogen, oxygen, phosphorus, and sulfur–arrange them in many different combinations, and what do you get?
In just the right combinations, you get life. Carbon, hydrogen, nitrogen, oxygen, phosphorus, and sulfur– the most basic of elements, but some of the most important. Together they can form countless combinations in organic compounds. And in just the right combinations, anything can happen.
Matter and Chemical Substances
Look around you. Everything is made of chemicals of one sort or another. The chair you are sitting in is made of atoms. The food you ate for lunch was built from atoms. Even the air you breathe is made of atoms. And you, as well as all living things, are made of matter. In fact, matter is the “stuff” of which all things are made. Anything that occupies space and has mass is known as matter. Matter, in turn, consists of chemical substances. A chemical substance is a material that has a definite chemical composition. It is also homogeneous, so the same chemical composition is found uniformly throughout the substance. A chemical substance may be an element or a chemical compound.
Life is chemistry organized into astonishing complexity and intricacy. To make sense of this organization we can look at life’s chemistry as a hierarchy, meaning levels of organization. From simple elemental ions to simple organic molecules, complexity rises with increasingly larger macromolecules.

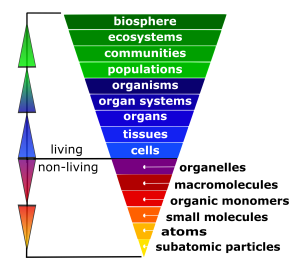
A person is between 1-2 meters (m) tall, but there are many length scales and biological levels of detail that are important for understanding biology. For perspective on size difference, consider an atom at 10-10 m. We can’t really grasp how small that is but try thinking big instead of small. A length of 1010 m is more than the distance from the Earth to the moon. The smallest length scale that we will cover is the size of subatomic particles.
The cell is the fundamental unit of life; therefore, you might think that your study of biology should begin with the cell. In fact, before you can truly understand how a cell functions, you must understand the building blocks from which cells are made. In the Introduction to Human Biology chapter, you learned that all life has a hierarchical organization. While cells are the first level of organization that displays all the properties of life, their component parts (from organelles down to subatomic particles) are not alive, as illustrated in the inverted pyramid in Figure 4.3.
To make sense of how cells work, you must constantly return to their parts (atoms and molecules) and the interactions among them. This is why a clear understanding of some chemistry is essential to understanding how life functions. This unit will focus on the atom. You will learn about the pieces that combine to form atoms, and how atoms combine to form different molecules, making up all the diverse matter you see around you. In this unit, you will explore only nonliving components of the hierarchy of life.
Learn By Doing 4.1
Decide which of the following correctly ranks the order of length scales from smallest to largest:
- insulin (a protein, which is a molecule) → carbon atom → pancreatic cells
- carbon atom → insulin (a protein, which is a molecule) → pancreatic cells
- pancreatic cells → carbon atom → insulin (a protein, which is a molecule)
- carbon atom → pancreatic cells → insulin (a protein, which is a molecule)
Hint: Insulin is a protein made of 51 amino acids, and each amino acid has about 20 atoms.
The first level of organization that is defined as living is:
- organelle
- macromolecule
- subatomic particle
- cell
Atoms: The Basic Unit of Matter
Learning Objectives
- Describe the structure of the atom.
- Differentiate between the terms “element“ and “atom.”
- Describe the location, mass, and charge of subatomic particles.
- Define electrostatic attraction and repulsion.
In this section, you will learn about the basic structure of the atom, the fundamental unit of matter. Living things are made up of atoms arranged in a complex and nonrandom way. This is one of the common features of all living organisms. The atom is the smallest level in the hierarchy of life that we will explore in this course. Understanding the properties of atoms allows us to predict and understand how atoms interact with one another to build molecules, such as hormones and DNA. In addition, an understanding of atoms allows us to predict how cells will react to different therapeutic drugs and toxins in the environment.
The Atom: Parts and Structure
All living and nonliving things are composed of matter. Matter can be defined as anything that occupies space and has mass. The mass of an object is a measure of how much matter it has (that is, how much “stuff” is in it). Mass is not exactly the same as weight, but here on Earth we can measure and compare the masses of different objects by weighing them.
When we explore matter scientifically, we find that it takes on many different structures as we zoom in at smaller and smaller scales. Everyday objects are mostly mixtures of molecules, which in turn are made up of atoms. Even air is a mixture of molecules. The atom is a good focal point for understanding matter; all matter is composed of atoms.
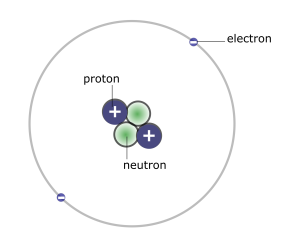
Atoms are unimaginably small. Even within a single microscopic cell, there is room for not just billions, but trillions or even hundreds of trillions of atoms. Amazingly, however, physicists now understand that most of the volume of an atom is actually made up of empty space. The atoms themselves are made of even smaller subatomic particles called protons, neutrons, and electrons. We cannot look at the parts of atoms with a microscope; they are simply too small. However, physicists have learned a great deal about atoms and subatomic particles through indirect methods. One way of modeling the atom is shown in Figure 4.4.
Atoms contain protons and neutrons, which are found in the nucleus (center) of the atom. Atoms also contain electrons, which are found outside the nucleus. Protons and electrons each have an electrical charge that influences how one atom will interact with another. Notice in the diagram of an atom to the left that the protons have a plus sign (+) indicating their positive electrical charge. Electrons have a negative charge designated by the negative sign (-). Opposite charges attract; protons and electrons are attracted to one another. Neutrons are electrically neutral, neither positive nor negatively charged. We will explore each subatomic particle in more detail below. You will want to know what changing the number of each type of subatomic particle means for any given atom.
Did I Get This? 4.1
1. Look at the beryllium atom in the drawing to the right. How many protons do you see in the nucleus of the atom?
Hint: Protons are found in the nucleus of the atom, which is in the middle.
2. Which of the following statements is true about electrons?
- Electrons are found in the nucleus.
- Electrons are positively charged particles.
- Both 1 and 2 are correct.
- Neither 1 nor 2 are correct.
3. What is the charge of a neutron?
- Neutrons have a negative charge.
- A neutron’s charge depends on the atom in which it is found.
- Neutrons have a positive charge.
- Neutrons have no charge.
4. What is the charge of the atom’s nucleus?
Elements
You’ve already seen that all atoms have a few structural characteristics in common. All atoms have a nucleus, are made up of protons and neutrons, and are surrounded by electrons. However, you are probably also aware that not all atoms are the same. In fact, atoms come in different types, called elements. There are 92 different kinds of elements that occur naturally on Earth. Each element has its own set of properties that are unique to atoms of its kind. Elements are designated by either one- or two-letter abbreviations and they are organized into a chart called the Periodic Table of Elements.
Elements differ from one another in the number of protons, electrons, neutrons, and chemical properties; these affect their ability to combine to form substances. An atom is the smallest unit of an element that retains all of the properties of that element. So, an element is a substance that is composed of a single type of atom. But, how do you know what kind of atom you have? It is easy! Count the number of protons in the atom’s nucleus, and that will tell you what kind of element that atom is. The number of protons in an atom is denoted by the atomic number displayed for each element in the Periodic Table.
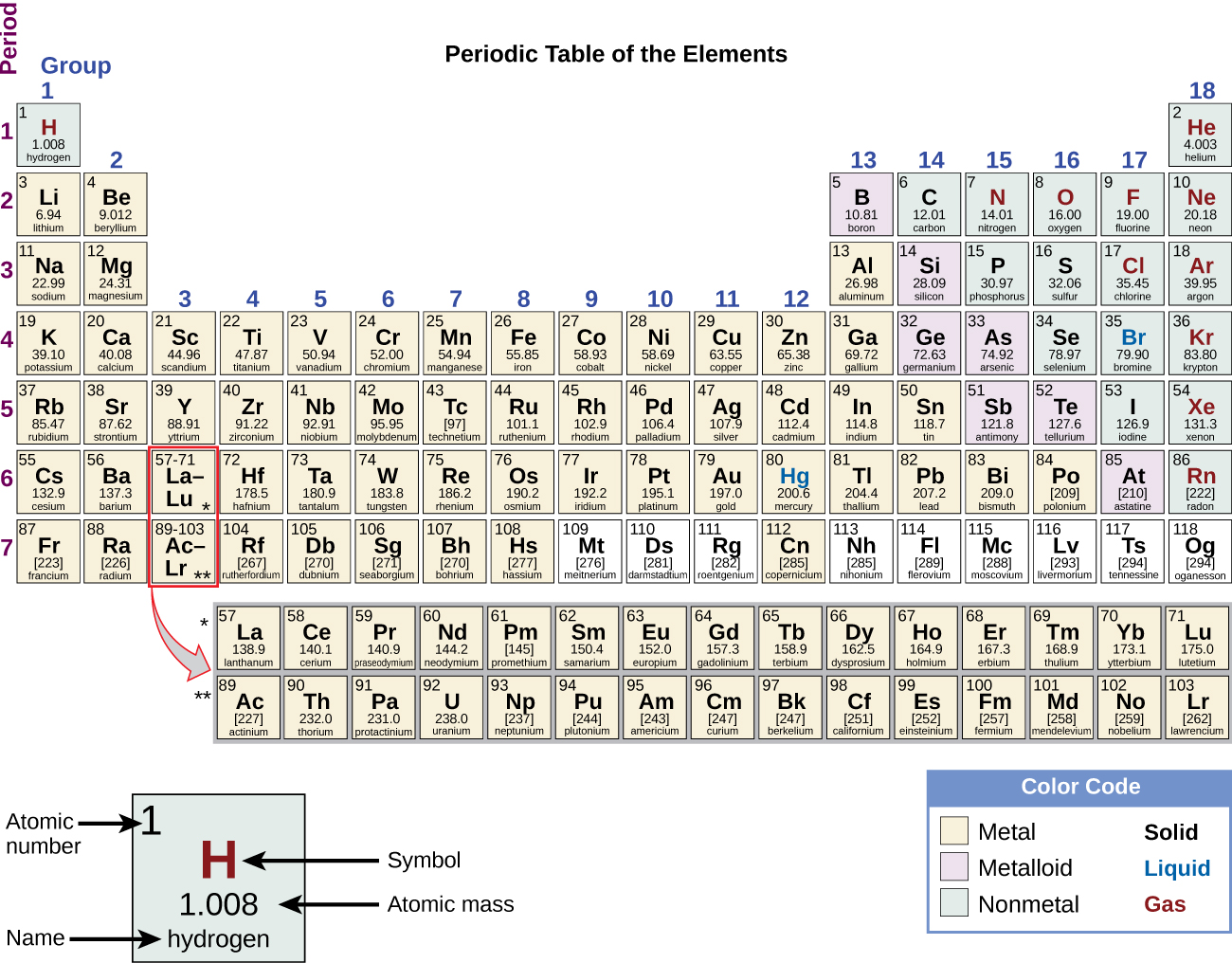
Learn By Doing 4.2
Look at the Periodic Table. All atoms of the element Hydrogen (H) have the same number of protons. How many protons do you think are in hydrogen atoms?
- 1.01, the atomic mass of hydrogen
- 1, the atomic number of hydrogen
- There is not enough information on the Periodic Table to answer this question.
- 1, because hydrogen is listed in the first row and first column of the Periodic Table.
How many protons are in the nucleus of a nitrogen (N) atom?
- 7.01
- 7
- 14.01
- 15
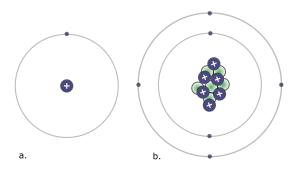
All hydrogen atoms contain one proton. In contrast, all carbon atoms contain six protons. It is only the number of protons found in the nucleus of the atom that determines the identity of an element. This number does not change if an atom participates in a chemical reaction. Only nuclear reactions generate enough energy to transform one element into another.
The hydrogen and carbon atoms shown to the right are displayed with a Bohr diagram. While this model oversimplifies atomic structure, it is a useful place to start when describing the structure of the atom. A Bohr diagram shows all subatomic particles and locates them correctly. We will discuss electron placement later in the chapter.
Elements of Life
Learning Objectives
- List the most common atoms (elements) found in biological systems.
- Interpret chemical formulas in terms of the number of atoms of each element present.
Element | Atmosphere (“Air”) | Earth’s Crust | Living Things |
Sulfur (S) | trace | trace | 0.2% |
Phosphorus (P) | trace | 0.1% | 1.1% |
Oxygen | 21% | 46% | 65% |
Nitrogen | 78% | trace | 3% |
Carbon | trace | trace | 18% |
Hydrogen | 0.1% | 46% | 10% |
All Other Elements | 1% | 7% | 2.7% |
total | 100% | 100% | 100% |
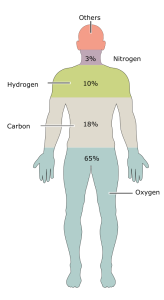
Some elements are found in relatively small amounts and are called “trace elements.” Examples include sodium (Na), potassium (K), chlorine (Cl), manganese (Mn), and Zinc (Zn). Throughout the course, you will see how atoms of these elements are very important to the functioning of cells, tissues, and organs.
The key biologically relevant elements are hydrogen (H), carbon (C), nitrogen (N), oxygen (O), phosphorous (P), and sulfur (S). These elements represent more than 95 percent of the mass of a cell, and all living things are made of cells and the molecules that cells make. A simple way to remember these 6 elements is the mnemonic “SPONCH.” Each letter represents the Periodic Table symbol for the element.
Carbon is a particularly important element since it is a major component of nearly all biological molecules. It is important to note that all of the elements present in living things are inorganic and non-living things, as we can see in Table 4.1. What makes the living different from the non-living is how atoms of these elements are organized.
Chemical Formulas
Molecules are the results of chemical reactions. Every molecule can be described with a chemical formula; a chemical formula is essentially an “ingredient list” for a molecule. Each formula tells you which ingredients (elements) are needed and how much of each is required (how many atoms of each element). Unlike an ingredient list, you might find in a cookbook, where the amount of each ingredient comes before its name, chemical formulas start with the element symbol and display the number of atoms as a subscript immediately afterward. Chemical formulas are also used as abbreviations for the names of compounds. For example, the formula for a single water molecule is written H2O, showing that the molecule is built with one oxygen atom and two hydrogen atoms. A subscript is used only when more than one atom of a given type is present.
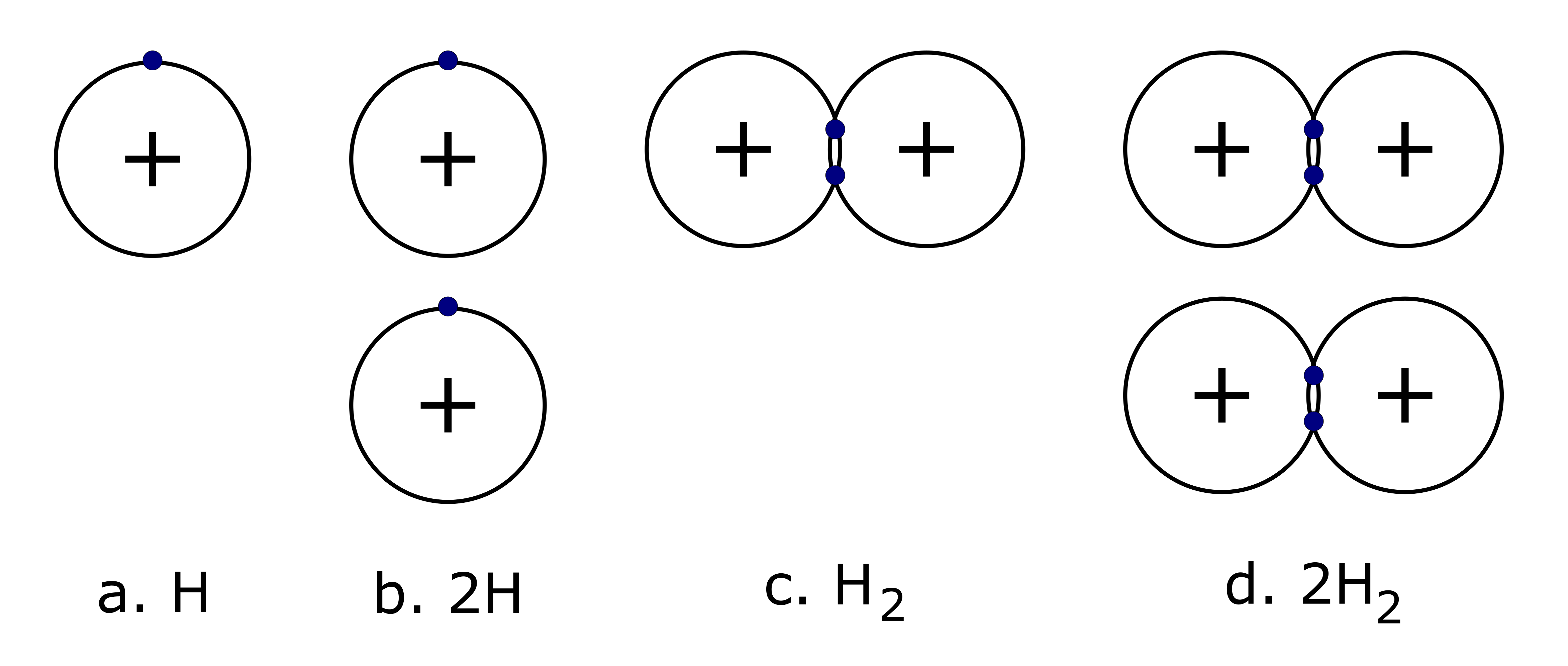
It is important to note that a subscript following a symbol and a number in front of a symbol do not represent the same thing; for example, H2 and 2H represent distinctly different things although 2 hydrogen atoms are present in both. H2 is a molecular formula; it represents a molecule of hydrogen gas, consisting of two atoms of the element that are chemically bonded together. The expression 2H, on the other hand, indicates two separate hydrogen atoms that are not combined as a unit. The expression 2H2 represents two molecules of hydrogen gas.
To calculate the mass of a single molecule, we find the mass of each individual atom in the molecule and add them together. For example, a water molecule (H2O) contains one oxygen atom that has a rounded mass of 16 amu (atomic mass units) and two hydrogen atoms that each have a rounded mass of one amu. Therefore, the mass of a water molecule is 16 amu + (2 x 1 amu) = 18 amu. If we want to know the mass of six molecules of water, we determine the mass of 1 molecule and multiply by 6. A coefficient is placed before the formula to indicate the number of molecules. Thus, 6 molecules of water are written 6H2O.
Learn By Doing 4.3
Which of the following elements are present in glucose?
- sulfur
- nitrogen
- oxygen
- calcium
How many carbon atoms are present in a single molecule of glucose? In two molecules?
What is the mnemonic used to remember the 6 elements that comprise most of our mass?
Subatomic Particles: Location, Mass, and Charge
Learning Objectives
- List the mass and charge of each subatomic particle
- Describe the structure of the atom
- Explain the structure of the atom in terms of electrostatic attraction and repulsion
- Determine the mass of a molecule using information from the Periodic Table.
Elements (atoms) are characterized by their atomic structure, which is made up of subatomic particles: protons, neutrons, and electrons. Protons and neutrons reside in the nucleus (center) of the atom and have a mass of one atomic mass unit (amu) each. Electrons are found outside of the nucleus, in 3-dimensional areas called orbitals that are organized into energy “shells.”
Particle | Location in Atom | Relative Mass (amu) |
Charge |
proton | nucleus | 1.0 | positive |
neutron | nucleus | 1.0 | no charge |
electron | cloud outside nucleus | negligible | negative |
The mass of an atom is called its atomic mass. When calculating atomic mass, we pay attention only to the protons and neutrons; the electrons have almost no mass. The atomic mass is the sum of the number of protons and the number of neutrons. By summing the atomic mass of all the atoms in a molecule, one can estimate the molecular mass of the molecule, which is expressed in atomic mass units (amu). Each of the heavy particles (neutron, proton) weighs one atomic mass unit, so a Helium (He) atom, which has two protons, two neutrons, and two electrons, weighs about four atomic mass units; that is, two protons plus two neutrons.
In addition to location and mass, protons and electrons have a property called “charge.” Charge can be “positive” or “negative.” Protons have a positive charge and neutrons have no charge, giving the nucleus a positive overall charge. Each electron has a negative charge that is equal in strength to the positive charge of a proton. Forces are exerted on charged objects by other charged objects. You’ve probably heard the saying “opposites attract,” which is true regarding charged particles. Opposite charges attract each other, a phenomenon called electrostatic attraction. Like charges repel each other, a phenomenon called electrostatic repulsion. Neutral objects have no influence on each other. This is illustrated in Figure 4.7. The forces of attraction and repulsion vary in strength based on the distance between charged particles and on the number of charges involved. (You may have learned this as Coulomb’s Law.) Electrons and the protons of the nucleus thus attract each other. Electrostatic attraction keeps the atom together, much like the force of gravity keeps the moon in orbit around Earth. An atom is electrically neutral if it has the same number of protons as electrons.

Atomic Number is the number of protons.
Atoms of different elements have many different features, including different sizes and levels of reactivity. For example, sodium is an incredibly reactive element, especially when it combines with water. Lead, on the other hand, is relatively inert. Yet all elements share some regular patterns that make it easier for us to categorize them and understand why they behave as they do.
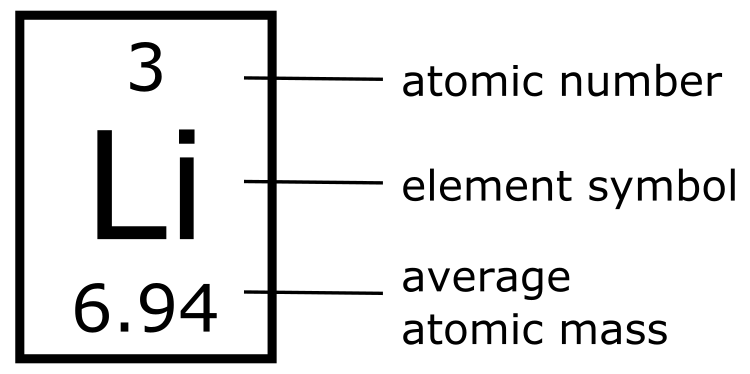
The only characteristic that truly identifies an element is its atomic number, which is the number of protons in each atom. The atomic number is constant and identical for all atoms of an element. For example, hydrogen (H) atoms always have only one proton and have an atomic number of one. If an atom has two protons, it has an atomic number of two, and it is helium (He). For this reason, if you started with one hydrogen atom and added a proton, the original hydrogen would now be helium. If an atom has three protons in its nucleus, it is a lithium (Li) atom. Beryllium (Be) has four protons in its nucleus, and so on. Based on their atomic numbers, elements can be organized into a Periodic Table of elements like the one pictured in Figure 4.5. The number at the top of each box in the table is the atomic number of the element.
The Periodic Table organizes elements based on atomic number, which is the smaller number in each square, often (but not always) placed above the element symbol. Each element has a unique chemical symbol, shown in the center of each square. Average atomic mass is usually shown below the element symbol.
Atomic Mass is the mass of the nucleus.
In addition to providing information about each element’s atomic number, most Periodic Tables also provide an average atomic mass for each element. The atomic mass, also known as the atomic weight, reports the mass of the nucleus. The mass of the nucleus is the sum of all protons and neutrons found in the nucleus. We refer to this as an atom’s mass number since the number of protons is interchangeable with their mass. The same is true for the number of neutrons and their mass. For example, if a carbon atom has six protons and six neutrons, it will have a mass number of 12. We can determine the number of neutrons if we know the mass number of an atom by subtracting the atomic number (the number of protons) from the atomic mass (the number of protons plus the number of neutrons. Using this information we can actually estimate the molecular mass of the molecule, which is then expressed in atomic mass units (amu) by summing the atomic mass of all the atoms in a molecule.
Learn By Doing 4.4
A mystery atom contains 7 protons, 7 electrons, and 7 neutrons.
- What is its mass? (Hint: The mass consists of the number of protons added to the number of neutrons. If the Periodic Table shows a decimal mass, we round to the nearest whole number unless we are given the number of neutrons and protons.)
- What is its charge? (Hint: The proton has a +1, the neutron has a zero charge, and the electron has a -1 charge.)
- Refer to the Periodic Table above. What element is this?
What happens when opposite charges get close to each other?
- They repel each other.
- They attract each other.
- Nothing happens.
Tryptophan is an essential amino acid that is used to build proteins and is modified to create certain hormones. Its 3-dimensional structure is important, but for now, we will focus on the atoms involved. The molecular formula is C11H12N2O2.
- Which of the following elements are present in tryptophan?
- sulfur
- nitrogen
- oxygen
- calcium
- How many carbon atoms are present in a single atom of tryptophan (C11H12N2O2)?
- What is the molecular mass of tryptophan in atomic mass units (amu)?
Electrons
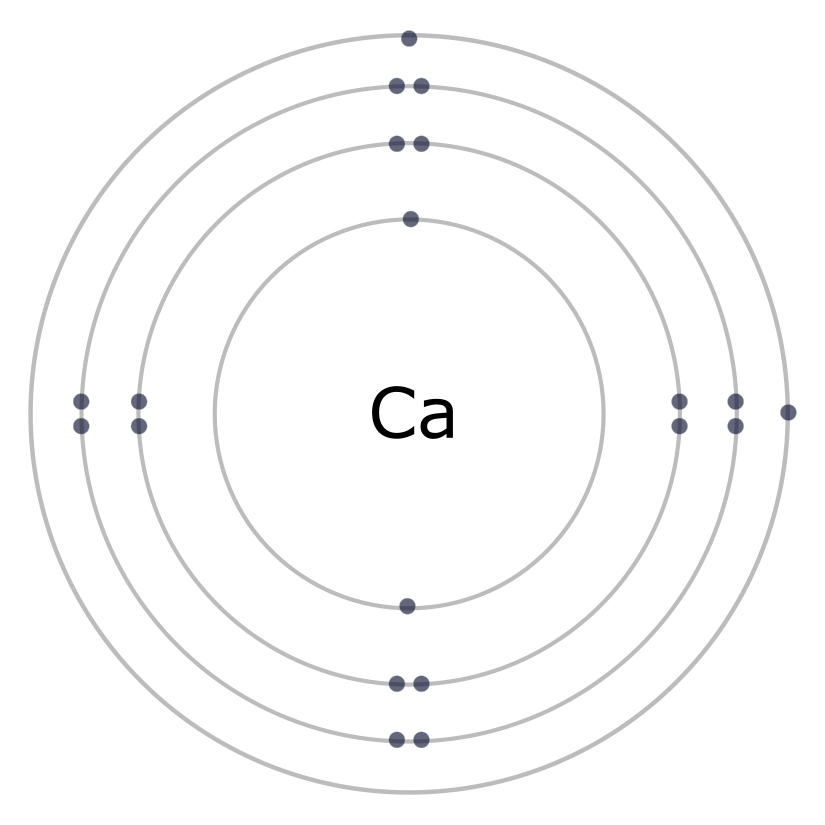
The electron is the reactive part of the atom. The number and location of electrons determine the character of interactions between atoms. As you recall, negatively charged electrons are pulled toward the positively charged nucleus. But electrons are kept in orbit by kinetic energy. Electrons that are close to the nucleus are less energetic than those electrons farther from the nucleus. Remember that the Periodic Table displays atoms that are electrically neutral? Another way of saying this is that the numbers of electrons and protons are equal and so the atom has no overall charge. This means that if you know the identity of an atom, (i.e., the number of protons) and you know the atom does not carry a charge, you can infer the number of electrons from the atomic number.
Electrons reside outside the nucleus and travel along 3-dimensional paths called orbitals. The orbitals are organized into regions called shells. There are specific rules for filling up the shells of the atom.
- The first shell (1n) can hold two electrons; the second (2n) and third (3n) shells can hold up to eight electrons.
- Electrons fill up inner shells before moving to the next higher shell. Why? The attraction force between positive and negative particles is stronger the closer they are together.
- The electrons that occupy the outermost shell are called valence electrons and are the electrons that are involved in chemical bonding. The chemical properties of an element depend mainly on the number of valence electrons.
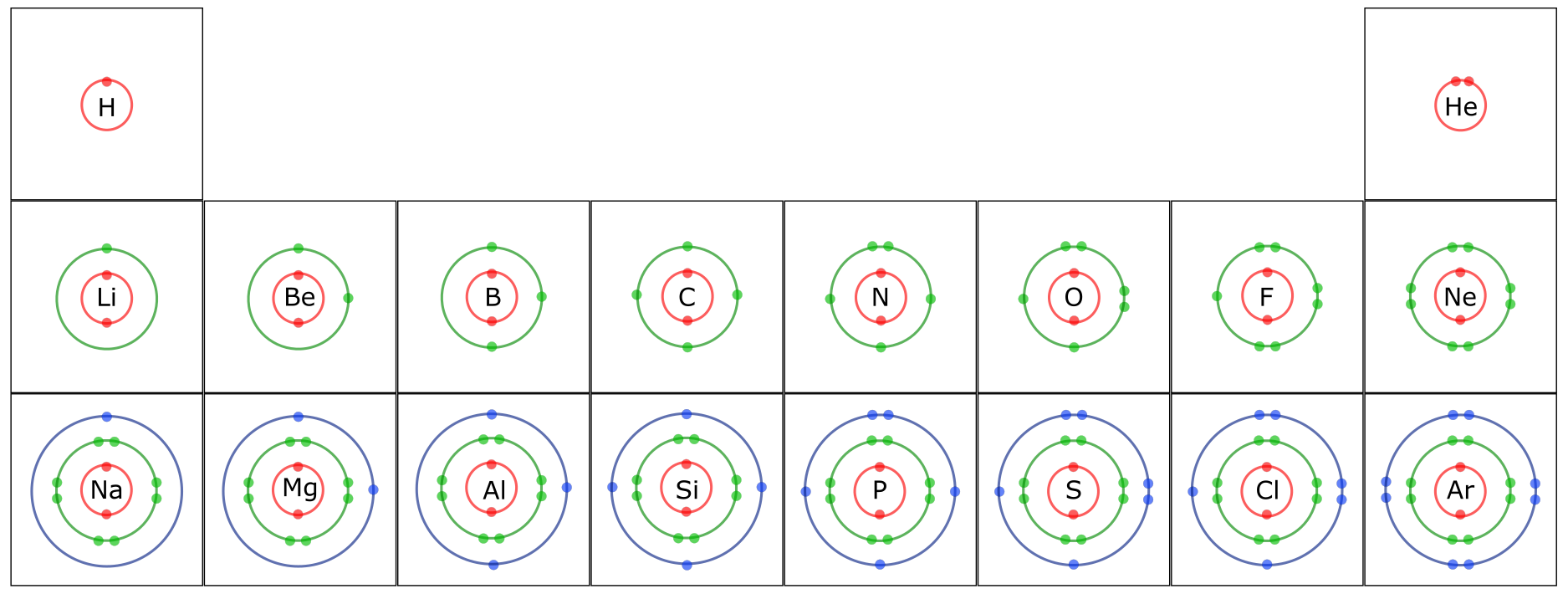
A key characteristic of atoms is that they are most “stable” when they have full outer electron shells. This is referred to as the “Octet Rule” since the valence shell of most atoms is full with 8 electrons. Helium (He) has a full outer shell with two electrons; directly below it in the Periodic Table is Neon. Neon (Ne) has an inner shell with two electrons and a full second shell with eight electrons. In fact, all of the elements in that column of the Periodic Table have full outer shells, are stable, and do not react with other elements.
Learn By Doing 4.5
Look up Fluorine (F) on the Periodic Table.
- How many protons does Fluorine have?
- How many electrons does Fluorine have?
- Is the following statement true or false? “All of a Fluorine atom’s electrons would be found in the first shell.”
Take a close look at Figure 4.12. What do you notice about the rows and columns?
Hint: Remember that you can assume that the number of electrons is the same as the number of protons.
Did I Get This? 4.2
Using the information provided on electrons, protons, and neutrons, explain how one can use the Periodic Table to determine the numbers of each of the subatomic particles in an atom.
Isotopes
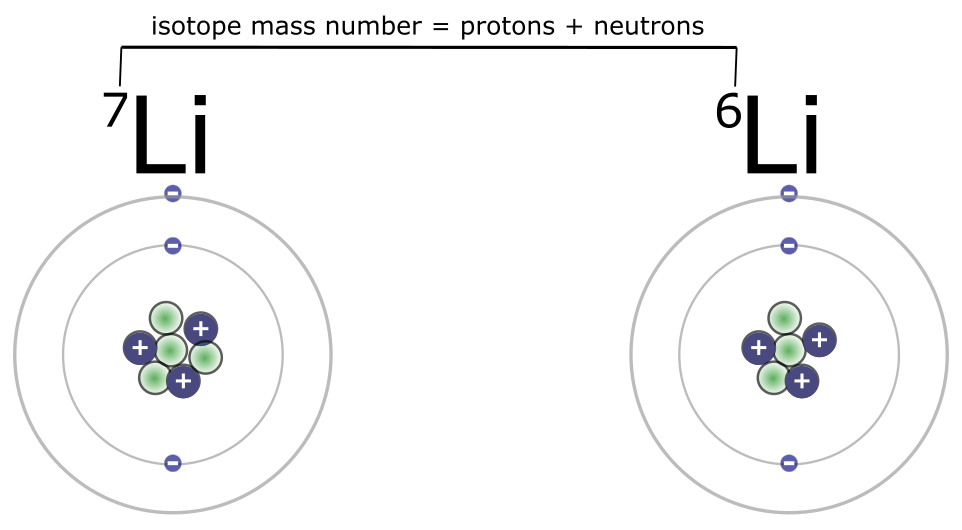
Notice that the atomic mass or atomic weight on the Periodic Table is not generally a whole number. For example, the atomic mass of carbon (C) is 12.01. How is that possible? Atoms cannot have fractions of protons or neutrons. The reason for the decimal mas is that not all atoms of an element have the same number of neutrons. For example, carbon atoms always have six protons; this is what defines an atom as carbon. But some carbon atoms have six neutrons, while others have seven or even eight neutrons. A carbon atom that has six neutrons would have an atomic mass of 12, a carbon atom with seven neutrons would have an atomic mass of 13, and a carbon atom with eight neutrons would have an atomic mass of 14. Atoms with the same atomic number but different atomic masses are called isotopes. Lithium provides another example, as shown in Figure 4.10. Most atoms of lithium have a mass number of 7 amu. Yet there are some atoms of lithium with a mass number of 6. These are both isotopes of the element lithium, although lithium 7 is more common. Taking into account lithium’s different isotopes and the greater prevalence of lithium-7, the weighted average value for the atomic mass of lithium is near 7: it’s listed at 6.94.
Scientists have learned that isotopes have many useful properties. Isotopes make useful tracers since all isotopes of an element participate in chemical reactions in the same way. When an isotope is radioactive, this means that it emits some energy (radiation) when it breaks down to a different isotope. For example, the radioactive isotope of iodine can be used to examine the human thyroid gland since the only area in the body where iodine is concentrated is in the thyroid gland. A small amount of Iodine-131 is administered; the release of gamma radiation can be “seen” by certain medical scanners and used to create an image of the patient’s thyroid.
Example: Medical Imaging
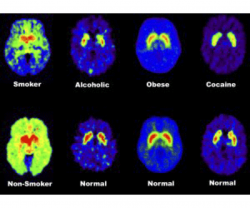
Radioactive isotopes can be used to visualize certain tissues for medical diagnosis. One example of this is radioactive iodine, which tends to concentrate in the thyroid gland. It emits radiation as it breaks down to another isotope of iodine. Special cameras can pick up this radiation and use it to create an image of the thyroid gland to determine if the patient’s thyroid is functioning normally. If there is abnormal activity in the thyroid gland, this can indicate cancer. Radioactive isotopes are also used in research. In positron-emission tomography (PET), a patient ingests sugar that is marked with a radioactive isotope. Cells that are using a lot of sugar (or need a lot of energy) will absorb more of the radioactive isotope. The patient is then moved into a PET scanner, where the radioactive isotopes are visualized and the machine translates the data into an image. PET scans have been used to study addiction. PET brain scans show chemical differences in the brain between those who have addictions and those who do not. The reference images in the bottom row come from those without addictions; the images in the top row come from patients with addiction disorders. These PET brain scans show that there are fewer than average dopamine receptors in the brains of those with addictions, so weaker dopamine signals are sent between cells.
Learn By Doing 4.6
Look up sodium on the Periodic Table.
- What is the atomic number of sodium (Na)? What is the atomic mass of sodium? (Round to the nearest whole number.)
- How many protons does sodium have?
- How many neutrons does sodium usually have?
Hint: Remember that: 1. No individual atom can have a decimal mass because there is no such thing as a partial proton or neutron, and 2. the mass of an atom is equal to the sum of its protons and neutrons.
The actual atomic mass on the periodic table for Sodium is 22.99, not 23. Why? Choose one of the following.
- Sodium actually has 11.99 neutrons.
- The actual atomic mass depends on the number of protons and that can be different in different atoms of the same element.
- Different atoms of sodium can have different numbers of neutrons. 22.99 is an average value.
Examine the image below.
- Which diagram represents Hydrogen with a mass number of 2?
Explain how you know.
Ions
Opposite charges cancel each other out when they are close together. So, what would you predict about the overall charge of an atom with an equal number of protons and electrons? That’s right — it is neutral. There is no charge; the atom will not be attracted to or repelled by charged objects. All atoms, in their elemental state, have equal numbers of protons and electrons, and for this reason, they have no net charge.
As you recall, an atom is most stable when it has a full outer shell. Some atoms achieve this endpoint by taking on extra electrons or giving them up. When this happens, the atom becomes an ion and it takes on an overall charge.
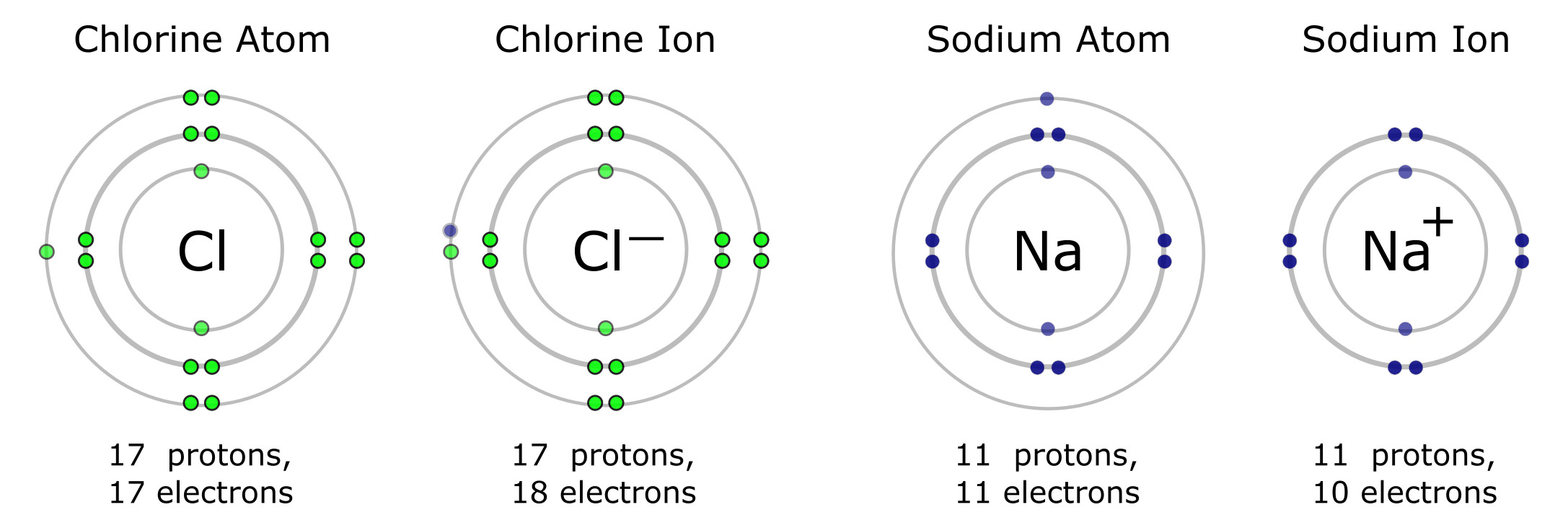
Some elements readily take on one or more extra electrons. They become anions that carry a negative charge because they have more electrons than protons. On the Periodic Table, elements toward the right (with the exception of the last column) are likely to take on extra electrons. For example, chlorine (Cl) has seven electrons in its valence shell. It is likely to take on one extra electron to fill its outer shell, becoming an anion with a charge of -1. The same can be said for other elements in the same column as chlorine. Conversely, elements on the left side of the periodic table tend to give up one or more electrons and become cations with a positive net charge. For example, sodium (Na) has one electron in its valence shell. It readily gives up this electron, becoming a cation with a charge of +1. The same can be said for lithium (Li) and other elements in this column.
Later in this chapter, we will explore how these electron transfers occur and will look at some of the other ways that atoms interact to achieve stable, full outer electron shells. In short, we’ll learn about chemical bonding.
Example: Ions and Human Health
Learn By Doing 4.7
Look up lithium (Li) on the periodic table. How would the electrons in Li fill the shells of the Li atom?
- Three electrons in the first shell
- One electron in the first shell and two electrons in the second shell
- All three electrons in the second shell
- Two electrons in the first shell and one electron in the second shell
If lithium gives up its third electron, the first shell of the atom is now the outer shell. This outer shell is full. What is the charge of this atom?
- +2
- +1
- -1
- -2
Hint: Compare the number of positively charged protons to the number of negatively charged electrons.
Look up magnesium (Mg) on the periodic table. Determine the number of electrons in the first, second, and third shells of a magnesium atom.
- 1st – 2, 2nd – 9, 3rd – 1
- 1st – 2, 2nd – 8, 3rd – 1
- 1st – 2, 2nd – 9, 3rd – 2
- 1st – 2, 2nd – 8, 3rd – 2
Mg gives up the two electrons in its third shell. This makes the second shell (which is full) the outer shell. What is the charge of Mg?
- +2
- +1
- -1
- -2
Did I Get This? 4.3
Imagine you have a magic wand that can change the number of any subatomic particle.
Describe how changing the number of each of the subatomic particles would alter an atom.
Chemical Bonding
Learning Objectives
- Define electronegativity.
- Discuss how atoms combine via ionic and covalent bonds to form compounds and molecules.
- Compare and contrast polar and non-polar covalent bonds.
- Compare hydrogen bonding with ionic and covalent bonding.
Chemical reactions occur when two or more atoms bond together to form molecules or when bonded atoms break apart. Scientists call the substances used at the beginning of chemical reactions reactants (usually on the left side of a chemical equation), and we call the substances at the end of the reaction products (usually on the right side of a chemical equation). It is important to remember that chemical reactions do not create or destroy atoms; the same number of atoms of each element are present in reactants and products. Chemical reactions involve the forming and breaking of connections between atoms (chemical bonds) and this leads to the rearrangement of atoms. We typically draw an arrow between the reactants and products to indicate the chemical reaction’s direction. For example, to create a water molecule, the chemical equation would be 2 H2 + O2 → 2 H2O.
An example of a simple chemical reaction is breaking down hydrogen peroxide molecules, each of which consists of two hydrogen atoms bonded to two oxygen atoms (H2O2). The reactant hydrogen peroxide breaks down into water molecules, each containing one oxygen atom bound to two hydrogen atoms (H2O), and oxygen, which consists of two bonded oxygen atoms (O2). In the equation below, the reaction includes two hydrogen peroxide molecules and two water molecules. This is an example of a balanced chemical equation, wherein each element’s number of atoms is the same on each side of the equation. According to the law of conservation of mass, the number of atoms before and after a chemical reaction should be equal, such that no atoms are, under normal circumstances, created or destroyed.
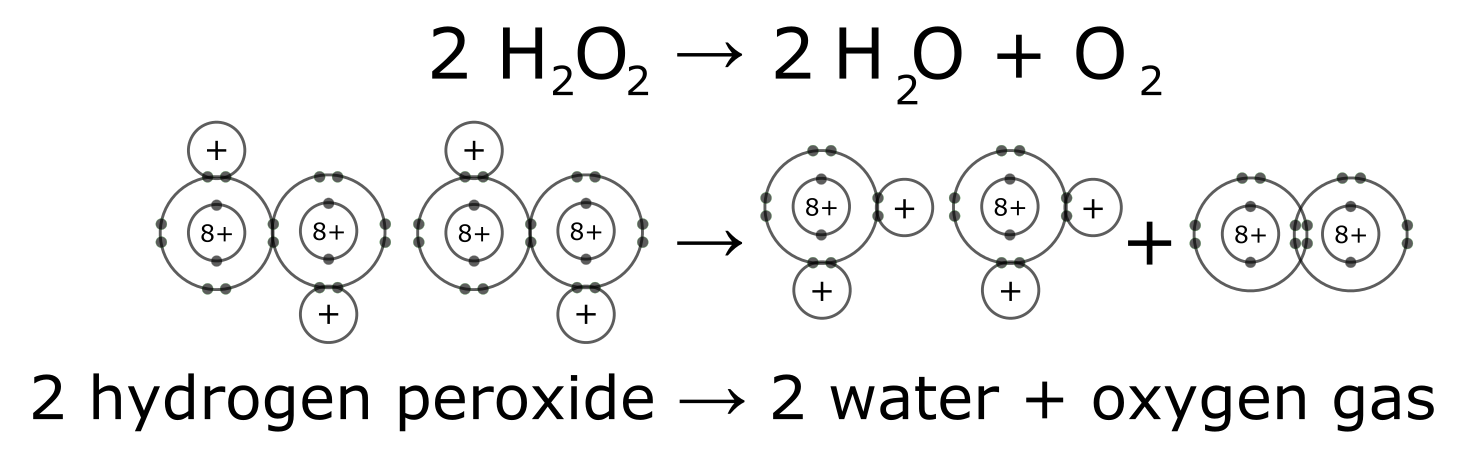
Chemical bonds result when atoms of the same element (e.g., carbon bound to carbon or C-C) or different elements (e.g., C-O, C-N, O-H) combine into relatively strong, usually electrically neutral, structures. There are two major types of chemical bonds: ionic and covalent. Covalent bonds can further be divided into polar covalent and nonpolar covalent bonds. A polar covalent bond is a type of covalent bond that results in a unique interaction between molecules. These polar bonds will interact with other polar bonds through an intermolecular attraction known as hydrogen bonding, such as that found between water molecules. Both the strong ionic and covalent chemical bonds and the weaker intermolecular forces such as hydrogen bonding are important in the functioning of the cell.
Did I Get This? 4.8
A molecule would best be described as:
- A stable bond between atoms of at least 2 different elements.
- A stable bond between 2 atoms of the same element.
- A charged molecule is made up of more than one atom.
- The first 2 responses are correct.
- All three responses are correct.
Hint: Molecules are commonly found in the environment because these are stable configurations.
The most stable situation for an atom is to have its outer shell completely filled with electrons. It is not easy to explain why this is true, but it is a rule of thumb that predicts how atoms will react with each other. Recall from the discussion on electrons, in the section above on atoms, that the first electron shell is full with two electrons, and the second and third shells are full with eight electrons. Atoms tend to bond to other atoms in such a way that both atoms have filled outer shells as a result of the interaction.
Electronegativity
The electronegativity of an element is the degree to which an atom will attract electrons in a chemical bond. Elements with higher electronegativities, such as N, O, and F (fluorine), have a strong attraction for electrons in a chemical bond and will, therefore, “pull” electrons away from less electronegative atoms. Elements with low electronegativity tend to “give away” electrons easily. Combinations of atoms with high and low electronegativities lead to the formation of ions and ionic bonds. Combinations of atoms with roughly equivalent electronegativity result in sharing of valence electrons, a bond referred to as a covalent bond.
As you can see in Figure 4.15, oxygen, nitrogen, and sulfur are electronegative atoms and represent the major electronegative atoms in biological molecules. Phosphorous, an important component in nucleic acids (e.g. DNA) is also electronegative.
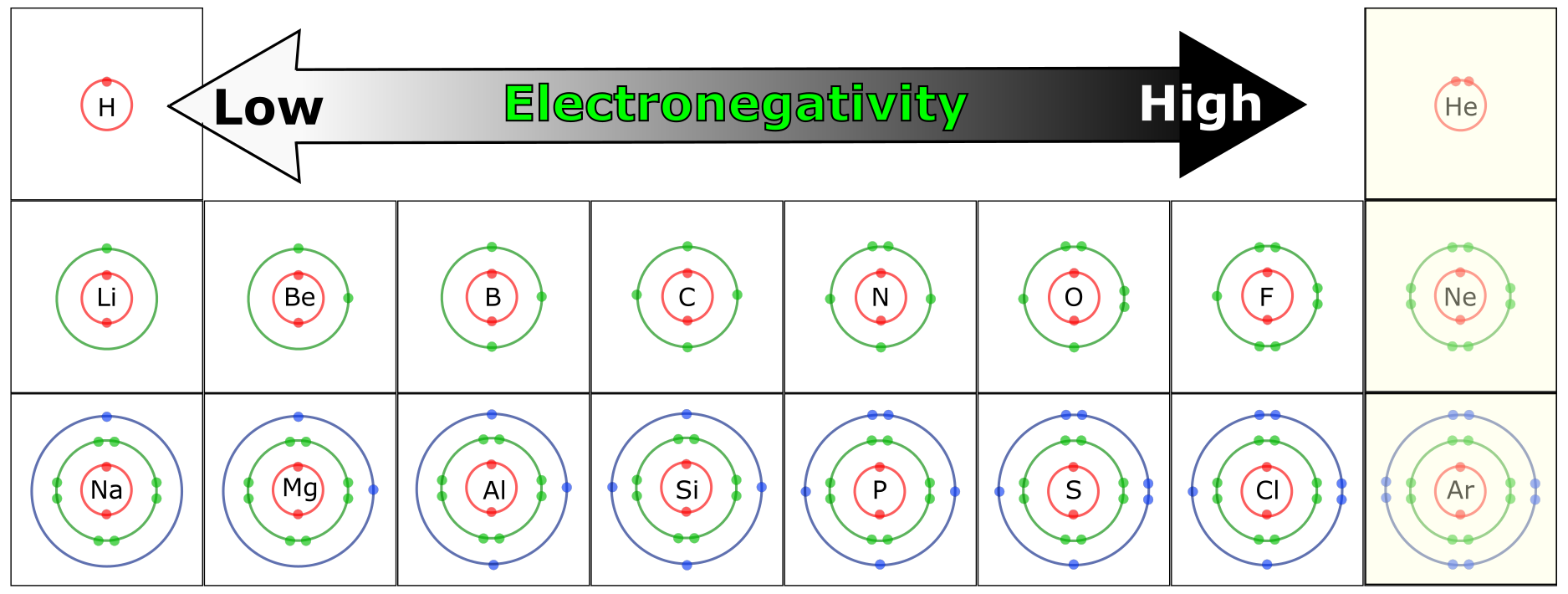
Did I Get This? 4.4
Define electronegativity in your own words.
Describe why electronegativity is important when thinking about chemical bonding.
Ionic Bonds
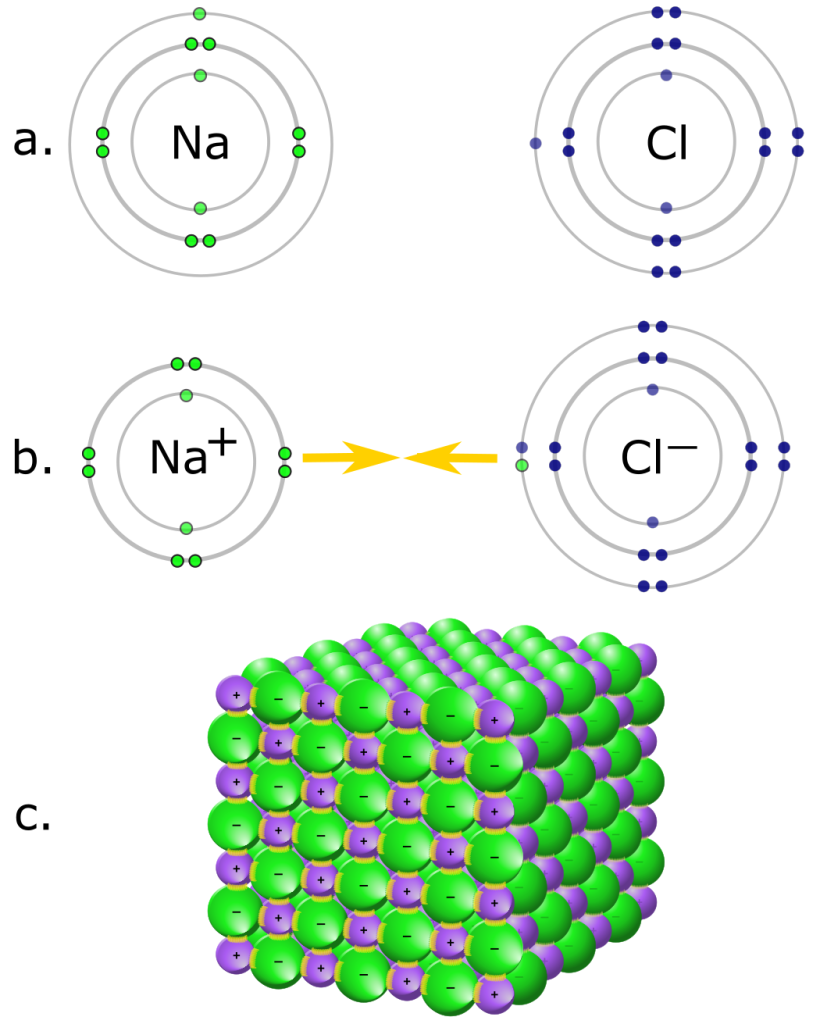
Changes to the nucleus of an atom require tremendous amounts of energy, so protons are not easily gained or lost by atoms. Electrons, on the other hand, are held loosely and can often be removed quite easily. When an object loses some electrons, the remaining object is now positively charged because it has an excess of protons. The electrons may either remain free or may attach to another object. In that case, the extra electrons cause that object to become negatively charged. Atoms that have lost electrons and become positively charged are called positive ions or cations, and atoms that have gained electrons and become negatively charged are called negative ions. or anions.
Ionic bonds are the result of electrostatic attraction between ions of opposite charges. Atoms form ions when they either take on or release electrons in order to fill their outer (valence) shell. Elements in the outer columns of the Periodic Table often react in this manner, becoming ions in order to achieve a full valence shell. For example, an element like sodium tends to lose its single valence electron when close to an element like chlorine, which gains an electron.
In fact, the reaction between sodium and chlorine is a great example of ionic bonding and produces a compound you have in your kitchen — table salt. Chlorine (Cl) has an atomic number of 17, so it has 7 electrons in its outermost shell. Chlorine needs one more electron to have a full outer shell.
Sodium (Na) has an atomic number of 11, with one electron in its outermost shell. Sodium needs to get rid of an electron, and then it will have a full outer shell. By losing an electron, sodium becomes a cation with a positive charge (+1).
By gaining an electron, chlorine becomes an anion with a negative charge (-1). Now each ion has a net charge and the two charges are opposite. The ions attract one another. The electrostatic interaction between the sodium ion and the chlorine ion is an ionic bond.
As discussed earlier, atoms with higher electronegativities tend to gain electrons and become anions, whereas those with lower electronegativities tend to lose electrons and become cations. The electrostatic attraction between a positively charged ion and a negatively charged ion is the basis of an ionic bond.
Learn By Doing 4.9
An ionic chemical bond is one in which:
- electrons are removed from one atom and transferred to another atom so that the two atoms become oppositely charged.
- outer shell electrons are shared by two atoms so as to satisfactorily fill the outer electron shells of both.
- protons or neutrons are shared by two atoms so as to satisfy the requirements of both.
- outer shell electrons of one atom are transferred to the inner electron shells of another atom.
Using the Periodic Table above, determine whether or not the pair of atoms listed will form an ionic bond.
- Li and Na
- K and Br
Covalent Bonds
Instead of transferring their electrons completely, atoms may remain in very close contact and share electrons so that a single valence shell is created. In essence, a shared electron is counted “twice” and participates in a larger shell that joins the two atoms. A single pair of shared electrons make a single covalent bond. Atoms can share two pairs of electrons (in a double bond), or even three pairs of electrons (in a triple bond). This sharing of electrons is called a covalent bond.
Two or more atoms held together by covalent bonds in a specified arrangement is called a molecule. The animation in Figure 4.19 illustrates the covalent bond that forms between two hydrogen atoms to form a molecule of hydrogen gas. Non-polar covalent bonds form between atoms of the same or similar electronegativities.
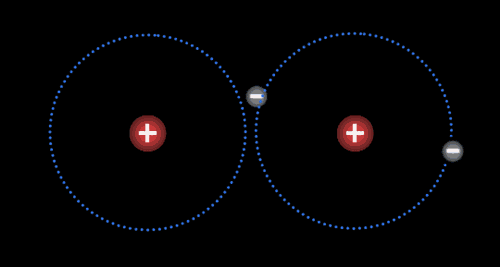
Each atom typically forms a specific number of covalent bonds when in a molecule with other atoms. The number of bonds that a particular atom will form is based on the atom’s valence electrons. Carbon for instance, which has four valence electrons, will form four bonds when it is in a molecule. Nitrogen, which has five valence electrons, will form three bonds, as is true in the ammonia molecule.
The number of bonds between two atoms, such as single bonds, double bonds, or triple bonds, helps determine the stability of the atomic interactions. Double bonds, which share two pairs of valence electrons between two atoms, are very strong. The strong bond of carbon double bonded to oxygen is found in amino acids (these will be discussed later). The number of valence electrons shared also controls the “shape” of the atomic interactions. Carbon double-bonded to oxygen forms a “flat” (planar) bond that does not rotate. This limits the shapes that the larger macromolecule, with repetitive double bonds, can form.
Learn By Doing 4.10
Oxygen has an atomic number of eight. How many electrons are in its outermost shell? 2, 4, 6, or 8?
How many covalent bonds can oxygen form to fill its outer shell? 1, 2, 3, 4, or 6?
How many hydrogen atoms will probably form covalent bonds with oxygen? 1, 2, 3, or 4?
Hints: The first shell contains two electrons. If there are a total of eight electrons and two are in the first shell, how many are left for the second shell?
What determines the number of covalent bonds formed by an atom?
Hint: Look at the outer electron shell in the Periodic Table and the Atomic Properties Table.
- Orbitals found in the atom.
- Electrons in each orbital in the atom.
- Electrons in the outer valence shell of the atom.
- Electron shells in the atom.
Representations of Molecular Structure
Learning Objectives
- Differentiate between Bohr model drawings and electron dot diagrams of the same molecules.
- Differentiate between structural and skeletal diagrams.
- Interpret drawings (structural and skeletal formulae) of molecules by identifying all atoms and covalent bonds in such drawings.
As you’ve learned, a chemical (molecular) formula is a representation of a molecule that uses chemical symbols to indicate the types of atoms followed by subscripts to show the number of atoms of each type in the molecule. This information can be used to determine how simple molecules are organized. We now introduce the types of molecular displays you will encounter in this course.
Bohr and Electron Dot Diagrams
By now you have a number of atoms represented with a Bohr diagram. A true Bohr model of any atom shows the number of all subatomic particles in the appropriate location in the atom. Diagram (b) below shows a modified Bohr diagram of the methane molecule that replaces the number of protons with the element symbol and does not provide information about the number of neutrons. (This is not a problem for 2 reasons: 1. the element symbol indicates the number of protons, and 2. isotopes of an element all participate in chemical bonding in the same way.)In fact, since only the number of electrons in the valence shell is important for bonding, chemists came up with a shorthand way to diagram covalently bonded atoms. Dot diagrams (or Lewis Dot Diagrams) show only the valence electrons for each atom and use the element symbol to represent the nucleus of each atom. A dot diagram of methane is shown in (c) below. Can you see the similarity between the modified Bohr diagram and the dot diagram?
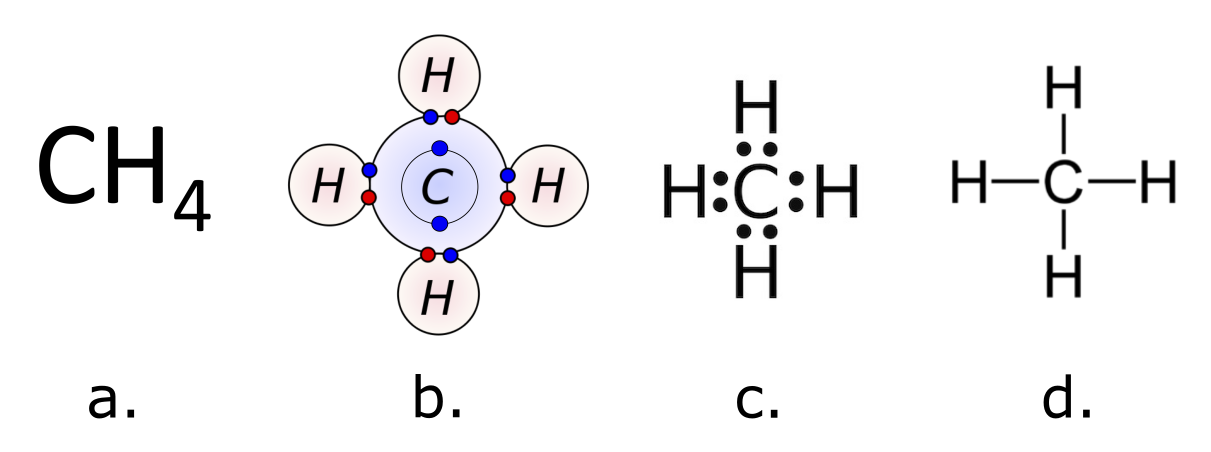
Molecular Formulas and Structural Diagrams
As you’ve learned, a molecular formula is a representation of a molecule that uses chemical symbols to indicate the types of atoms followed by subscripts to show the number of atoms of each type in the molecule. The structural formula for a compound gives the same information as its molecular formula (the types and numbers of atoms in the molecule) but also shows how the atoms are connected in the molecule. The structural formula for methane, (d) above, contains symbols for one C atom and four H atoms, indicating the number of atoms in the molecule. The lines represent bonds that hold the atoms together. You might have guessed that each line represents a pair of shared electrons. A single covalent bond is drawn as one line; a double covalent bond is drawn as 2 lines. Each atom is represented by its element symbol. Notice that the carbon in methane has formed four covalent bonds with four hydrogen atoms. Because carbon has four electrons in its outer shell, it can always form four covalent bonds with other elements.
Over time the way in which molecules are modeled has changed. Each molecular representation has advantages and disadvantages, but each contributes to our ability to understand how molecules interact. Table 4. below shows four different models used for showing the molecule for butane (C4H10).
Type of Model | Representation |
Lewis structures are two-dimensional representations of molecules that illustrate each atom as its chemical symbol. Lines indicate bonds to other atoms, and non-bonding electrons are represented as small dots next to the chemical symbols. | ![]() |
Skeletal diagrams show selected atoms as their chemical symbols while depicting some carbon atoms as corners between lines and leaving out hydrogen atoms that are assumed to be in the structure. This type of notation is used mainly in organic chemistry (chemistry associated with living things). | ![]() |
Ball and stick models are three-dimensional models where atoms are represented by spheres of different colors, and bonds are represented by sticks between the spheres. Particular atoms are associated with different colors; for example, black is usually used to represent carbon, and white to represent hydrogen. | ![]() |
Space-filling models are similar to ball and stick models in that they are three-dimensional models that represent atoms as colored spheres. The difference between them is that instead of sticks representing bonds, as in the ball and stick model, the spheres that represent atoms are fused together, and the size of the sphere represents the size of the actual atom. |
![]() |
The information above is from “Chemical Structures—Visualizing the Invisible,” University of Hawaii, Manoa. |
Learn By Doing 4.11
Examine the molecule to the right.
- What type of diagram is this?
- Carbon atoms are represented by black spheres. How many carbon atoms are present?
- Hydrogen atoms are represented by pale gray spheres. How many hydrogen atoms are present?
- Oxygen atoms are represented by red spheres. How many oxygen atoms are present?
- What is the chemical formula for this molecule?
- What do the lines between spheres represent?
- What is the mass of a single molecule of this substance?
Water has Polar Covalent Bonds
Learning Objectives
- Differentiate between polar and non-polar covalent bonds.
- Describe why hydrogen bonds are referred to as intermolecular forces.
Water is the basis of life. Without it, life is not possible. Water accounts for up to 75 percent of the human body’s weight. Water provides a relatively stable medium in which chemical reactions can take place. Water’s unique chemical properties (as a solvent with a high boiling point and high heat capacity) make it essential for homeostasis. The body’s thermoregulation relies on water’s high heat capacity to buffer it against swings in external temperature. Cooling of the body is carried out by evaporative water loss—perspiration. Water is also vital as a transport medium. Oxygen is carried by red blood cells suspended in serum, which is mostly water. Nutritional substances are dissolved in water and transported to cells. Water is used to dissolve and dilute waste molecules. If the body is deprived of water for very long, death will result.
Water has several properties that contribute to its suitability to support life as we know it. Most of these properties are a result of the fact that water is a polar molecule. Oxygen is more electronegative than hydrogen and draws the electrons that it shares in the covalent bond towards itself. Because water is polar, the partially positive end of one water molecule will be attracted to the partially negative end of a neighboring water molecule. This attraction is called a hydrogen bond. Hydrogen bonding occurs between partially negatively charged atoms with high electronegativity—oxygen, nitrogen, or fluorine—and partially positively charged hydrogen atoms that are bonded to oxygen, nitrogen, or fluorine atoms.
In a molecule such as molecular hydrogen (H2), the electrons are shared equally because each atom has the same electronegativity. However, in some molecules, one atom is more electronegative than another, in which case the electrons are not shared equally. For example, one oxygen atom is covalently bonded to two hydrogen atoms in a water molecule. Because an oxygen atom is more electronegative than a hydrogen atom, the oxygen atom draws the electrons being shared toward itself and away from the less electronegative hydrogen. atoms unequally share the electrons and are attracted more to one nucleus than the other. Because of the unequal electron distribution between the atoms of different elements, a slightly positive (δ+) or slightly negative (δ–) charge develops. (Note that the δ symbol allows us to distinguish partial and full charge.)
When electrons in a covalent bond are shared in an unequal manner, it is termed a polar, or polar covalent, bond. This unequal sharing of electrons results in the more electronegative element, in this example, the oxygen atom, having a slightly negative charge, and the less electronegative element, in this example, the hydrogen atom, having a slightly positive charge.
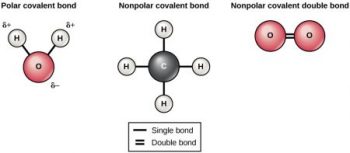
Molecules with polar bonds have characteristics of both ionic and covalent bonds. Water is an excellent example of a polar molecule, and as you probably know is necessary for life. The macromolecules found in cells often have atoms that differ in electronegativity, so polar bonds are often present in organic molecules. Whether or not a molecule is polar has significant implications on how that molecule interacts with other molecules and ions in biological systems.
Hydrogen bonds
Hydrogen bonding is a force that acts between molecules; it is an intermolecular attraction rather than an intramolecular force like a covalent bond. Hydrogen bonds are critical to cellular homeostasis. They are the principal force that helps to build and maintain the three-dimensional structure of proteins, carbohydrates, and nucleic acids. The overall stability of these molecules is due in part to the cumulative effect of the large number of hydrogen bonds present. Hydrogen bonds are found in and between a variety of molecules. For example, the enormous number of hydrogen bonds between strands of plant cellulose provides the strength and structure of the plant cell wall. As another example, wool (sheep hair) has lots of proteins with an enormous number of hydrogen bonds that provide the curly structure of individual wool fibers. These curly fibers trap air spaces which makes wool such a good insulator. When washed at high temperatures, these hydrogen bonds are broken, and the wool fibers will lose their shape, probably damaging any wool clothing.
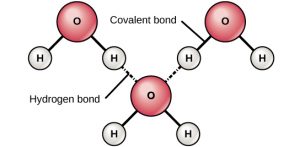
The electronegative oxygen (red) draws electrons to it, creating 2 areas of partial negative charge on oxygen and a partial positive charge on each hydrogen atom.
A water molecule attracts four other water molecules toward itself. One molecule to each of the two free pairs of electrons in the oxygen atom valence shell and one to each of the hydrogen atoms covalently bonded to the oxygen. As the water molecules associate with each other, they have a defined structure dictated by the tetrahedral geometry of the electrons around the oxygen atom. The partially positive hydrogen atoms are attracted to the free electron pairs from other water molecules while the partially negative charge on the oxygen’s free electron pairs is attracted to the partially positive hydrogen atoms from another water molecule.
Example: Solid Water
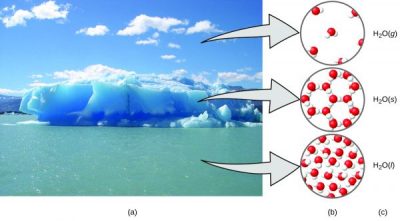
(a) Moisture in the air, icebergs, and the ocean represent water in the macroscopic domain. (b) At the molecular level (microscopic domain), gas molecules are far apart and disorganized, solid water molecules are close together and organized, and liquid molecules are close together and disorganized. (c) The formula H2O symbolizes water, and (g), (s), and (l) symbolize its phases.
One excellent illustration of hydrogen bonding is the change in the hydrogen bonding of water in ice. These stabilized bonds give ice its crystal-like appearance. The diagram above shows the network-like formation of weak bonds between molecules. This type of hydrogen bonding also helps large protein structures stabilize.
Learn By Doing 4.12
Describe the difference between polar and non-polar covalent bonds.
Describe the similarity between polar and non-polar covalent bonds.
Which statement is true about hydrogen bonds?
- Hydrogen bonds attract molecules or parts of molecules to each other.
- All of these answers are correct.
- Hydrogen bonds are formed between oxygen and hydrogen atoms in a water molecule.
- Hydrogen bonds connect atoms to each other, forming molecules.
Hint: Hydrogen bonds are not the same as covalent bonds.
Hydrogen bonds form in biological systems between molecules that contain hydrogen and what other molecule(s)?
- oxygen
- nitrogen
- carbon
- oxygen and nitrogen
Hints: The more electronegative atom will attract the electrons more. Which atom attracts the electrons more?
Energy Associated with Bonds
Each of the bond types has a measurable amount of energy. To break a bond, the equivalent amount of energy must be expended. In metabolism, bonds are broken in molecules, such as glucose, to “release” energy.Energy is not actually released when bonds break. It is released when new bonds form. Only if we consider a reaction as a whole can we imply that breaking bonds "releases" energy. The cell utilizes this energy to drive other energy-consuming reactions. Covalent bonds have the most energy associated with them. Imagine that, on average, a covalent bond has approximately contains 100 units of energy. In contrast, ionic bonds, hydrogen bonds, and hydrophobic interactions have approximately five units of energy associated with each of them. (It should be noted here that throughout this course, approximations will be used for certain values to make estimations as we move to more complex systems.)
Joining atoms together builds more complex and larger molecules than the individual atoms that compose them. The table below illustrates some similarities and differences among ionic, covalent, and hydrogen bonds.
Type of Bond | |||
Covalent Bond | Ionic Bond | Hydrogen Bond | |
Energy Associated with Bond in an Aqueous Environment | 100 units | 5 units | 5 units |
Characteristics of Bond | Electron pairs are shared, creating a strong bond between atoms | Electrons transfer from one atom to another, resulting in a moderately strong electrostatic attraction between oppositely charged ions | Weak electrostatic attraction between molecules with areas of partial charges |
Participants in Bond | Atoms with similar electronegativities | Atoms with differing electronegativities | Molecules with polar covalent bonds |
Inter- or intramolecular? | Intramolecular: Creates molecule | Intramolecular: creates a compound | Intermolecular: Attraction between 2 molecules or between 2 parts of the same large molecule |
The non-covalent bonds that have been introduced have approximately 20 times less energy associated with them and, thus are more easily broken individually. However, hydrogen bonds generally form extensive networks, and the total energy associated with the network is the sum of the individual interactions. As anyone who has done a "belly flop" into a swimming pool knows, breaking a large surface area of water is extremely difficult (and painful).
Learn By Doing 4.13
As you've learned, there are different kinds of chemical bonds-- ionic, covalent, and hydrogen.
Close your eyes and imagine you are so tiny that you can swim around inside a cell. You see lots of molecules bouncing into one another as they interact with the water molecules inside the cell. You notice that molecules formed with a certain kind of bond seem to be more stable in the aqueous environment of our cells. Which type of bond is it? Why is that type of bond the most stable?
Did I Get This? 4.5
The partial negative charge at one end of a water molecule is attracted to the partial positive charge of another water molecule. This attraction is called:
- a hydration shell
- a hydrogen bond
- an ionic bond
- a covalent bond
- a hydrophobic bond
Which bonds must be broken for water to vaporize (become a gas)?
- hydrogen bonds
- ionic bonds
- hydrophobic interactions
- nonpolar covalent bonds
- polar covalent bonds
Inorganic Molecules in the Cell
Learning Objectives
- Differentiate between organic and inorganic molecules.
- Describe the physiologically important properties of water.
An inorganic compound is a substance that does not contain both carbon and hydrogen. Many inorganic compounds contain hydrogen atoms, such as water (H2O) and the hydrochloric acid (HCl) produced by your stomach. In contrast, only a handful of inorganic compounds contain carbon atoms. Carbon dioxide (CO2) is one of the few examples.
An organic compound, then, is a substance that contains both carbon and hydrogen. Organic compounds are synthesized via covalent bonds within living organisms, including the human body. Recall that carbon and hydrogen are two of the most abundant elements in your body. In the next chapter, you will discover how these two elements combine in the foods you eat, the compounds that make up your body structure, and the chemicals that fuel your functioning.
The following section examines the three groups of inorganic compounds essential to life: water, salts, acids, and bases. Organic compounds are covered in detail in the next chapter.
Water
As much as 75 percent of an adult’s body weight is water. This water is contained both within the cells and between the cells that make up tissues and organs. Its several roles make water indispensable to human functioning.
Water as a Lubricant and Cushion
Water is a major component of many of the body’s lubricating fluids. Just as oil lubricates the hinge on a door, water in synovial fluid lubricates the actions of body joints, and water in pleural fluid helps the lungs expand and recoil with breathing. Watery fluids help keep food flowing through the digestive tract and ensure that the movement of adjacent abdominal organs is friction-free.
Water also protects cells and organs from physical trauma, cushioning the brain within the skull, for example, and protecting the delicate nerve tissue of the eyes. Water cushions a developing fetus in the mother’s womb as well. This cushioning action is possible because water is cohesive. The term cohesion is used to refer to molecules that are attracted to themselves. One water molecule is attracted to another due to the two slightly positive (δ+) and two slightly negative (δ–) areas of charge. In the case of water, cohesion results from hydrogen bonding between water molecules.
Water as a Heat Sink
A heat sink is a substance or object that absorbs and dissipates heat but does not experience a corresponding increase in temperature. In the body, water absorbs the heat generated by chemical reactions without greatly increasing in temperature. Moreover, when the environmental temperature soars, the water stored in the body helps keep the body cool. This cooling effect happens as warm blood from the body’s core flows to the blood vessels just under the skin and is transferred to the environment. At the same time, sweat glands release warm water in sweat. As the water evaporates into the air, it carries away heat, and then the cooler blood from the periphery circulates back to the body's core.
Water has high specific heat. The term refers to the amount of energy that must be added to a substance to raise its temperature by one degree Celsius. Thus water is a heat sink because it has high specific heat. This, in turn, results from the tremendous number of hydrogen bonds in water. Many of these bonds must be disrupted to raise the water's temperature.
Water as a Solvent
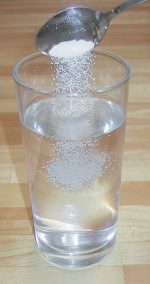
A mixture is a combination of two or more substances, each of which maintains its own chemical identity. In other words, the substances in the solution are not chemically bonded into a new, larger chemical compound. The concept is easy to imagine if you think of powdery substances such as flour and sugar; when you stir them together in a bowl, they obviously do not bond to form a new compound. The room air you breathe is a gaseous mixture containing three elements as molecules—nitrogen, oxygen, and argon—and one compound, carbon dioxide. There are three types of liquid mixtures, all of which contain water as a key component. These are solutions, colloids, and suspensions.
For cells in the body to survive, they must be kept moist in a water-based liquid called a solution. In chemistry, a liquid solution consists of a solvent that dissolves a substance called a solute. An important characteristic of solutions is that they are homogeneous; the solute molecules are distributed evenly throughout the solution. If you were to stir a teaspoon of sugar into a glass of water, the sugar would dissolve into sugar molecules separated by water molecules. The ratio of sugar to water in the left side of the glass would be the same as the ratio of sugar to water in the right side of the glass. If you were to add more sugar, the ratio of sugar to water would change, but the distribution—provided you had stirred well—would still be even.
Because of this, water is considered the “universal solvent” in biology, and it is believed that life cannot exist without water. Water is certainly the most abundant solvent in the body; essentially, all of the body’s chemical reactions occur among compounds dissolved in water. Because water molecules are polar, with regions of positive and negative electrical charge, water readily dissolves ionic compounds and polar covalent compounds. Such compounds are referred to as hydrophilic or “water-loving.” As mentioned above, sugar dissolves well in water. This is because sugar molecules contain regions of hydrogen-oxygen polar bonds, making them hydrophilic. Non-polar molecules, which do not readily dissolve in water, are called hydrophobic or “water-fearing.”
Concentrations of Solutes
Various mixtures of solutes and water are described in chemistry. The concentration of a given solute is the number of particles of that solute in a given space. For example, oxygen gas makes up about 21 percent of atmospheric air). In the bloodstream of humans, glucose concentration is usually measured in milligrams (mg) per deciliter (dL), and in a healthy adult averages about 100 mg/dL.
A colloid is a mixture that is somewhat like a heavy solution. The solute particles consist of tiny clumps of molecules large enough to make the liquid mixture opaque (because the particles are large enough to scatter light). Familiar examples of colloids are milk and cream. Thyroid hormone is stored in the thyroid glands as a thick protein mixture, also called a colloid.
A suspension is a liquid mixture in which a heavier substance is suspended temporarily in a liquid but, over time, settles out. This separation of particles from a suspension is called sedimentation. An example of sedimentation occurs in the blood test that establishes sedimentation rate, or sed rate. The test measures how quickly red blood cells in a test tube settle out of the watery portion of blood (known as plasma) over a set period of time. Rapid sedimentation of blood cells does not normally happen in the healthy body, but aspects of certain diseases can cause blood cells to clump together, and these heavy clumps of blood cells settle to the bottom of the test tube more quickly than normal blood cells.
Learn By Doing 4.14
The polar character of water that results in its ability to form extensive hydrogen bonding networks gives rise to several properties of water that are important to sustaining life. The following is a list of a few of these properties. Explain why each of these properties is important to sustaining life.
- Water is cohesive and has high surface tension.
- Ice is less dense than water.
- Much heat energy is required for the vaporization of water (a large amount of heat is required to convert liquid water to a gas).
- Water has a high specific heat capacity (a large amount of heat is required to raise the temperature of one gram of water one degree Celsius).
- Water has very polar covalent bonds.
Hint: You may want to think about what would happen if a certain property were different.
How do biologists and chemists define the term "organic"?
The Role of Water in Chemical Reactions
Learning Objective
Two types of chemical reactions involve the creation or consumption of water: dehydration synthesis and hydrolysis. We will explore these further in the next chapter. They are included here
In dehydration synthesis, one reactant gives up an atom of hydrogen and another reactant gives up a hydroxyl group (OH) in the synthesis of a new product. In the formation of their covalent bond, a molecule of water is released as a byproduct. This is also sometimes referred to as a condensation reaction.
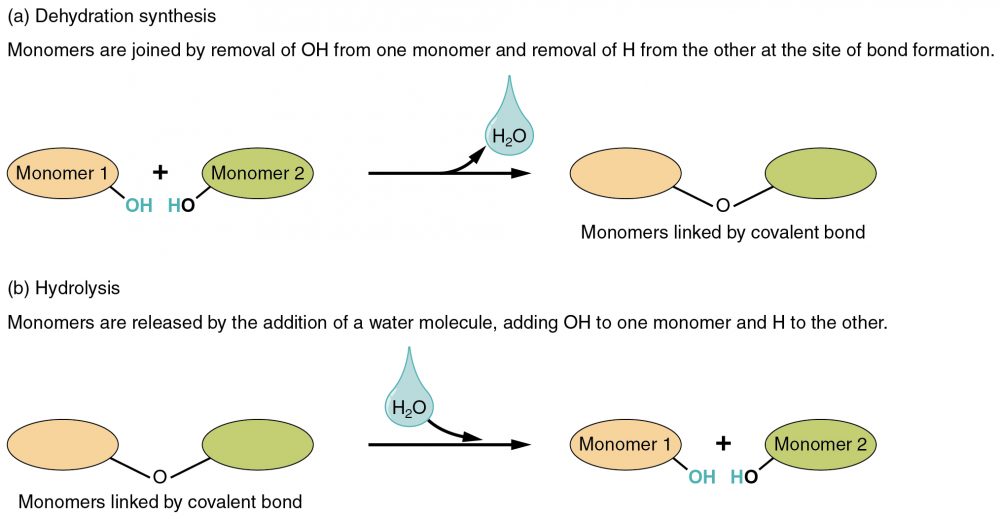
In hydrolysis, a molecule of water disrupts a compound, breaking its bonds. The water is itself split into H and OH. One portion of the severed compound then bonds with the hydrogen atom, and the other portion bonds with the hydroxyl group.
These reactions are reversible and play an important role in the chemistry of organic compounds.
Did I Get This? 4.6
Describe dehydration synthesis and hydrolysis.
Salts and Electrolytes
Learning Objectives
- Define the terms salt and electrolyte.
- Describe the meaning of "spheres of hydration."
Salts are formed when ions form ionic bonds. In these reactions, one atom gives up one or more electrons and thus becomes positively charged, whereas the other accepts one or more electrons and becomes negatively charged. Electrostatic attraction then draws the oppositely charged ions together. A salt is a substance that, when dissolved in water, dissociates into ions other than H+ or OH–. This fact is important in distinguishing salts from acids and bases, discussed next.
The attraction between oppositely charged ions in an ionic compound is strong. However, because of the polarity of water, when many ionic compounds are in aqueous solutions, they become dissociated into ions. For instance, when an ionic compound such as table salt (NaCl) is dissolved in water, it separates into Na+ and Cl- ions. The water molecules surround the ions to form polar interactions such that the positive ends of the water molecules are arranged around negative ions, and the negative ends of the water molecules surround positive ions, pulling the ions away from one another. The ions become encapsulated by water spheres, which are called spheres of hydration. Ions are very important in the biological world. Spheres of hydration are important in a cell because they maintain the separation of the many ions of the cell from each other. The sphere of hydration must be broken in order for binding to take place with a specific binding partner.
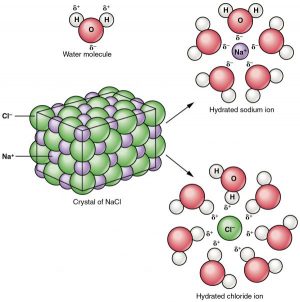
As a typical salt, NaCl dissociates completely in water. The positive and negative regions on the water molecule (the hydrogen and oxygen ends, respectively) attract the negative chloride and positive sodium ions, pulling them away from each other. Whereas non-polar and polar covalently bonded compounds break apart into molecules in solution, salts dissociate into ions. These ions are electrolytes capable of conducting an electrical current in a solution. This property is critical to the function of ions in transmitting nerve impulses and prompting muscle contraction.
Many other salts are important in the body. For example, bile salts produced by the liver help break apart dietary fats, and calcium phosphate salts form the mineral portion of teeth and bones.
Did I Get This? 4.7
- Differentiate between the terms salt and electrolyte.
- Describe the meaning of "spheres of hydration."
Acids, Bases, and pH
Learning Objectives
- Describe how acids and bases are defined in terms of ions.
- Interpret numbers on the pH scale in terms of whether the number describes a substance is acidic, neutral, or basic.
Acids and bases, like salts, dissociate in water into electrolytes. Acids and bases can very much change the properties of the solutions in which they are dissolved.
An acid is a substance that releases hydrogen ions (H+) in solution. Because an atom of hydrogen has just one proton and one electron, a positively charged hydrogen ion is simply a proton. This solitary proton is highly likely to participate in chemical reactions. Strong acids are compounds that release all of their H+in solution; that is, they ionize completely. Hydrochloric acid (HCl), which is released from cells in the lining of the stomach, is a strong acid because it releases all of its H+ in the stomach’s watery environment. This strong acid aids in digestion and kills ingested microbes. Weak acids do not ionize completely; some of their hydrogen ions remain bonded within a compound in solution. An example of a weak acid is vinegar, or acetic acid; it is called acetate after it gives up a proton.
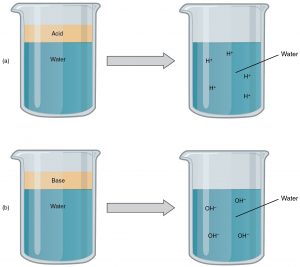
A base is a substance that releases hydroxyl ions (OH–) in solution or one that accepts H+ already present in solution. The hydroxyl ions (also known as hydroxide ions) or other basic substances combine with H+ ions to form a water molecule, thereby removing H+ and reducing the solution’s acidity. Strong bases release most or all of their hydroxyl ions; weak bases release only some hydroxyl ions or absorb only a few H+. Food mixed with hydrochloric acid from the stomach would burn the small intestine, the next portion of the digestive tract, after the stomach if it were not for the release of bicarbonate (HCO3–), a weak base that attracts H+. Bicarbonate accepts some of the H+protons, thereby reducing the acidity of the solution.
The Concept of pH
A solution's relative acidity or alkalinity can be indicated by its pH. A solution’s pH is the negative, base-10 logarithm of the solution's hydrogen ion (H+) concentration. The log conversion reduces a tenfold change in hydrogen ion concentration to a one-unit change in pH. The minus sign changes the negative numbers that would be obtained from log(H+) to positive ones. Since the pH scale is an inverse scale, the concentration of protons is high at low pH and low at high pH. As an example, a pH 4 solution has an H+ concentration that is ten times greater than that of a pH 5 solution. That is, a solution with a pH of 4 is ten times more acidic than a solution with a pH of 5.
The concept of pH will begin to make more sense as you study the pH scale, like that shown below. The scale consists of a series of increments ranging from 0 to 14. A solution with a pH of 7.0 is considered neutral—neither acidic nor basic. Pure water has a pH of 7. The lower the number below 7, the more acidic the solution, or the greater the concentration of H+. The concentration of hydrogen ions at each pH value is 10 times different than the next pH. The higher the number above 7, the more basic (alkaline) the solution, or the lower the concentration of H+. Human urine, for example, is ten times more acidic than pure water, and HCl is 10,000,000 times more acidic than water. A solution's hydrogen ion concentration (H+) is important because biological systems contain functional groups whose properties are changed by changes in the hydrogen ion concentration.
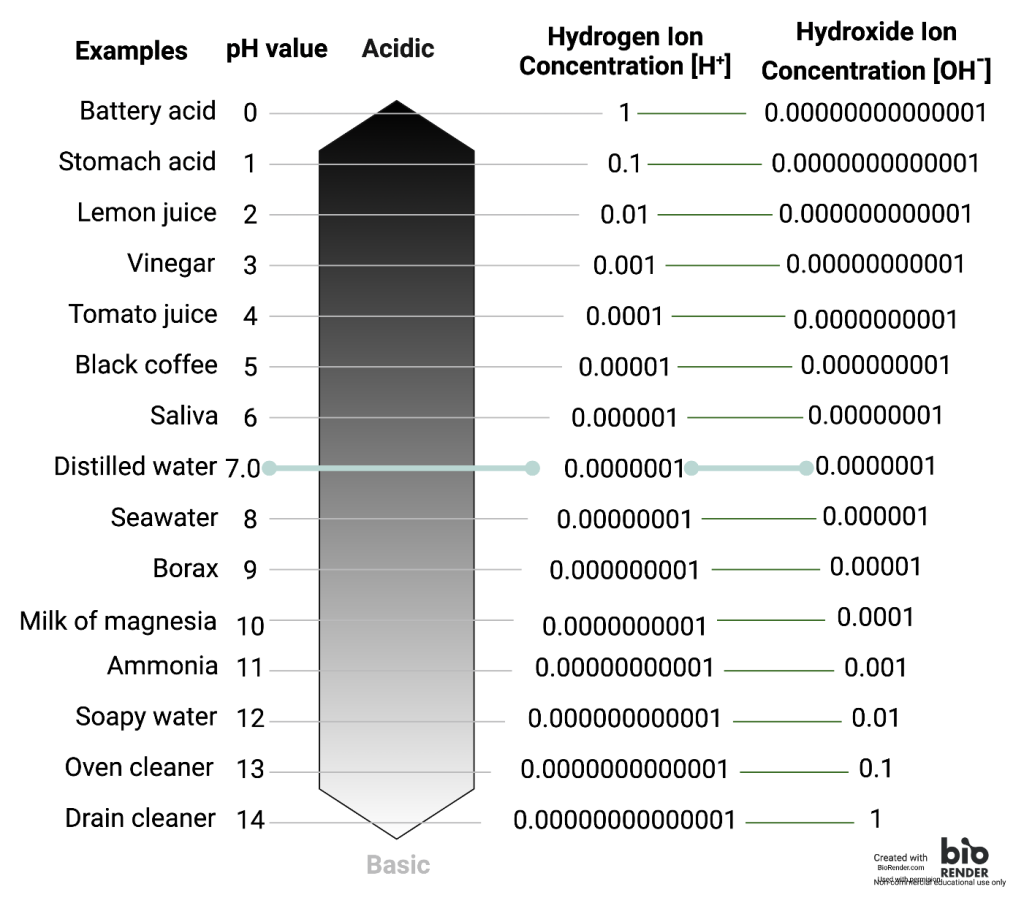
Sea water has a pH around 8, and lemon juice has a pH of 2. Which has the greatest number of free protons in solution?
- Sea water
- Lemon juice
Hint: Refer to the pH scale in the text. Observe that as pH increases, free proton concentration [H+] decreases. Remember as pH increases, the concentration of H+ ions decreases.
The difference in pH between lemon juice and seawater is six orders of magnitude. Written out another way, that represents what difference in proton concentration?
- a six-fold difference
- a difference of 1 x 106
- a difference of 0.06
Hint: Orders of magnitude are 10-fold differences. Refer to the scale and count the orders of magnitude between pH 2 and pH 8.
Did I Get This? 4.8
The pH of a solution is a measure of ________________ in the solution.
- the concentration of electrons
- the molecular weight of ions
- total of all ions
- the concentration of H+ ions
Blueberries grow best in moderately acidic soils. Which of the following would be an appropriate pH of the soil in which blueberries can be expected to grow well?
- 1.2
- 7.0
- 12.0
- 4.8
- 8.3
Buffers
Living organisms are quite sensitive to even small changes in pH. The chemical reactions of life are tuned to a specific and often narrow pH range; outside this range, the reactions will not proceed normally. Therefore, organisms have mechanisms that work to maintain a constant pH level. This is true both at the cellular level and at the level of the whole organism. Maintaining a stable pH in a living organism is important to homeostasis. Homeostasis refers to the ability of an organism (or cell) to maintain stable internal conditions despite constantly changing environmental conditions.
The pH of human blood normally ranges from 7.35 to 7.45, although it is typically identified as pH 7.4. At this slightly basic pH, blood can reduce the acidity resulting from the carbon dioxide (CO2) constantly being released into the bloodstream by the trillions of cells in the body. We'll discuss this later in the course. Homeostatic mechanisms (along with exhaling while breathing) normally keep the pH of blood within this narrow range. This is critical because fluctuations—either too acidic or too alkaline—can lead to life-threatening disorders.
All cells of the body depend on homeostatic regulation of acid-base balance at a pH of approximately 7.4. The body, therefore, has several mechanisms for this regulation, involving breathing, the excretion of chemicals in urine, and the internal release of chemicals collectively called buffers into body fluids. A buffer is a solution of a weak acid and its conjugate base. A buffer can neutralize small amounts of acids or bases in body fluids. For example, if there is even a slight decrease below 7.35 in the pH of a bodily fluid, the buffer in the fluid—in this case, acting as a weak base—will bind the excess hydrogen ions. In contrast, if pH rises above 7.45, the buffer will act as a weak acid and contribute hydrogen ions.
Example: Homeostatic Imbalances
Excessive acidity of the blood and other body fluids is known as acidosis. Common causes of acidosis are situations and disorders that reduce the effectiveness of breathing, especially the person’s ability to exhale fully, which causes a buildup of CO2 (and H+) in the bloodstream. Acidosis can also be caused by metabolic problems that reduce the level or function of buffers that act as bases, or that promote the production of acids. For instance, with severe diarrhea, too much bicarbonate can be lost from the body, allowing acids to build up in body fluids. In people with poorly managed diabetes (ineffective regulation of blood sugar), acids called ketones are produced as a form of body fuel. These can build up in the blood, causing a serious condition called diabetic ketoacidosis. Kidney failure, liver failure, heart failure, cancer, and other disorders also can prompt metabolic acidosis.
In contrast, alkalosis is a condition in which the blood and other body fluids are too alkaline (basic). As with acidosis, respiratory disorders are a major cause; however, carbon dioxide levels fall too low in respiratory alkalosis. Lung disease, aspirin overdose, shock, and ordinary anxiety can cause respiratory alkalosis, which reduces the normal concentration of H+.
Metabolic alkalosis often results from prolonged, severe vomiting, which causes a loss of hydrogen and chloride ions (as components of HCl). Medications also can prompt alkalosis. These include diuretics that cause the body to lose potassium ions, as well as antacids when taken in excessive amounts, for instance, by someone with persistent heartburn or an ulcer.
Learn By Doing 4.16
Complete the following sentence: A buffer is...
In your own words, describe why buffers are critical to homeostasis in living things.
Chapter Summary
An atom contains a very small nucleus composed of positively charged protons and uncharged neutrons, surrounded by a much larger volume of space containing negatively charged electrons. The nucleus contains the majority of an atom’s mass because protons and neutrons are much heavier than electrons, whereas electrons occupy almost all of an atom’s volume.
The number of protons in the nucleus of an atom is its atomic number. This is the defining trait of an element: Its value determines the identity of the atom. An atom's total number of protons and neutrons is called its mass number. The number of neutrons is, therefore, the difference between the mass number and the atomic number. The mass of one atom is usually expressed in atomic mass units (amu), which is referred to as atomic mass.
A neutral atom must contain the same number of positive and negative charges, so the number of protons equals the number of electrons. Therefore, the atomic number also indicates the number of electrons in an atom. When the numbers of these subatomic particles are not equal, the atom is electrically charged and is called an anion. An atom's charge is defined as Atomic charge = number of protons − number of electrons.
The average masses of most elements are not whole numbers because most elements exist naturally as mixtures of two or more isotopes. Isotopes of an element are atoms with the same atomic number but different mass numbers; isotopes of an element, therefore, differ from each other only in the number of neutrons within the nucleus.
Electrons can be donated or shared between atoms, creating molecules through the formation of ionic and covalent bonds, respectively. These are intramolecular forces.
Inorganic compounds essential to human functioning include water, salts, acids, and bases. These compounds are inorganic; that is, they do not contain both hydrogen and carbon. Water is a lubricant and cushion, a heat sink, a component of liquid mixtures, a byproduct of dehydration synthesis reactions, and a reactant in hydrolysis reactions. Salts are compounds that, when dissolved in water, dissociate into ions other than H+ or OH–. In contrast, acids release H+ in solution, making it more acidic. Bases accept H+, thereby making the solution more alkaline (caustic).
The pH of any solution is its relative concentration of H+. A solution with pH 7 is neutral. Solutions with pH below 7 are acids, and solutions with pH above 7 are bases. A change in a single digit on the pH scale (e.g., from 7 to 8) represents a ten-fold increase or decrease in the concentration of H+.
The pH of a solution is a measure of the concentration of hydrogen ions in the solution. A solution with high hydrogen ions is acidic and has a low pH value. A solution with a high number of hydroxide ions is basic and has a high pH value. The pH scale ranges from 0 to 14, with a pH of 7 being neutral. Buffers are solutions that moderate pH changes when an acid or base is added to the buffer system. Buffers are important in biological systems because of their ability to maintain constant pH conditions.
Water has many properties that are critical to maintaining life. It is polar, allowing for the formation of hydrogen bonds, which allow ions and other polar molecules to dissolve in water. Therefore, water is an excellent solvent. The hydrogen bonds between water molecules give water the ability to hold heat better than many other substances. As the temperature rises, the hydrogen bonds between water continually break and reform, allowing for the overall temperature to remain stable, although increased energy is added to the system. Water’s cohesive forces allow for the property of surface tension. All of these unique properties of water are important in the chemistry of living organisms.
*
"Learn By Doing" and "Did I Get This?" Feedback
Learn By Doing 4.1
Decide which of the following correctly ranks the order of length scales from smallest to largest:
- insulin (a protein, which is a molecule) → carbon atom → pancreatic cells
No: Atoms and ions are smaller than proteins. Insulin is made up of more than a thousand atoms. - carbon atom → insulin (a protein, which is a molecule) → pancreatic cells
Yes: Insulin is 44x larger than calcium and pancreatic beta cells are 4000x larger than insulin. - pancreatic cells → carbon atom → insulin (a protein, which is a molecule)
No: Cells are the largest of these choices. - carbon atom → pancreatic cells → insulin (a protein, which is a molecule)
No: Cells are larger than atoms, but cells are much larger than proteins.
The first level of organization that is defined as living is:
- organelle
No: Components of living things by themselves are not considered living. - macromolecule
No: Macromolecules are found in living things but they are not living. - subatomic particle
No: This is the first level of organization, but subatomic particles are not living. - cell
Yes, The cell is the smallest unit of life.
Did I Get This? 4.1
1. Look at the beryllium atom in the drawing to the right. How many protons do you see in the nucleus of the atom? 2, 3, 4, 5, or 9?
Hint: Protons are found in the nucleus of the atom, which is in the middle.
- 2 protons: No, the protons are found in the nucleus, which is in the middle of the atom.
- 3 protons: No, All the protons are the same color in the middle of the atom
- 4 protons: Yes, there are 4 protons, which are blue, in the middle of the atom.
- 5 protons: No, neutrons are the green particles in the nucleus. The blue particles are the protons.
- 9 protons: No, protons and neutrons are in the nucleus but only the blue particles are protons.
2. Which of the following statements is true about electrons?
- Electrons are found in the nucleus.
No: Electrons are found orbiting around in the nucleus. - Electrons are positively charged particles.
No: Electrons are negatively charged particles. - Both 1 and 2 are correct.
No: Electrons are not passively charged particles and they are not found in the nucleus. - Neither 1 nor 2 are correct.
Yes: Electrons are negatively charged particles and they are found orbiting the nucleus.
3. What is the charge of a neutron?
- Neutrons have a negative charge.
No: Neutrons are not negatively charged. - A neutron's charge depends on the atom in which it is found.
No: The charge of a neutron is always the same, no matter what kind of atom it is found in. - Neutrons have a positive charge.
No: Neutrons are not positively charged. - Neutrons have no charge.
Yes: Neutrons are neutral, meaning that they carry no charge.
4. What is the charge of the atom's nucleus?
The nuclei of all atoms are positively charged; the presence of neutrons does not counter the positive charge of the protons. The positively charged nucleus attracts negatively charged electrons.
Learn By Doing 4.2
Look at the Periodic Table. All atoms of the element Hydrogen (H) have the same number of protons. How many protons do you think are in hydrogen atoms?
- 1.01, the atomic mass of hydrogen
No: The atomic mass approximates the weight of the atom, not the number of protons. - 1, the atomic number of hydrogen
Yes: The atomic number is equal to the number of protons in the nucleus of an atom. - There is not enough information on the Periodic Table to answer this question.
No: Everything you need to know to answer this question can be found on the Periodic Table. - 1, because hydrogen is listed in the first row and first column of the Periodic Table.
No: Not all the elements in the first row or first column have one proton.
How many protons are in the nucleus of a nitrogen (N) atom?
- 7.01
No: An atom cannot have 0.01 protons; that is, every proton has a mass of 1 amu; there are no protons with fractional masses. The number of protons (and neutrons) in a single atom is always a whole number.
- 7
Yes: The atomic number of nitrogen is 7, indicating that the nitrogen atom has 7 protons. - 14.01
No: An atom cannot have 0.01 protons. This number approximates the weight or amount of the nitrogen atom. - 15
No: Even though nitrogen is found within column 15, this does not tell you the number of protons in the nucleus.
Learn By Doing 4.3
Glucose has the chemical formula C6H12O6. It is produced by plants from carbon dioxide and water during the reactions of photosynthesis. It is also the primary source of energy for our cells.
Which of the following elements are present in glucose?
- sulfur
- nitrogen
- oxygen
- calcium
How many carbon atoms are present in a single molecule of glucose? In two molecules? 6 carbon atoms in one molecule, 12 carbon atoms in 2 molecules
Using information from the table above, calculate the molecular mass of a single molecule of glucose in atomic mass units (amu).
carbon: 6 atoms with a mass of 12 amu = 72 amu
hydrogen: 12 atoms with a mass of 1 amu = 12 amu
oxygen: 6 atoms with a mass of 16 amu = 96 amu
In total, the mass of this molecule equals 72 + 12 + 96 = 180 amu
What is the mnemonic used to remember the 6 elements that comprise most of our mass?
SPONCH, which stands for Sulfur, Phosphorus, Oxygen, Nitrogen, Carbon, and Hydrogen.
Learn By Doing 4.4
A mystery atom contains 7 protons, 7 electrons, and 7 neutrons.
- What is its mass? (Hint: The mass consists of the number of protons added to the number of neutrons.)
14 amu, the combined mass of neutrons and protons
- What is its charge? (Hint: The proton has a +1, the neutron has a zero charge, and the electron has a -1 charge.)
This atom has no charge since the number of positively charged protons is equivalent to the number of negatively charged electrons.
- Refer to the periodic table. What element is this?
Nitrogen, since this atom has 7 protons. The atomic number of nitrogen is 7, and the atomic number is the number of protons.
What happens when opposite charges get close to each other?
- They repel each other.
- They attract each other.
Opposite charges attract one another and like charges repel.
- Nothing happens.
Learn By Doing 4.5
Look up Fluorine (F) on the Periodic Table.
- How many protons does Fluorine have? 9 protons
- How many electrons does Fluorine have? 9 electrons
- Is the following statement true or false? "All of a Fluorine atom's electrons would be found in the first shell."
False, electron shells are filled from the nucleus outward and the innermost shell can only accommodate only 2 electrons. Since Fluorine has 9 electrons, there must be 2 energy shells, with 2 electrons in the first shell and 7 electrons in the second.
Take a close look at Figure 4.12. What do you notice about the rows and columns?
Hint: Remember that you can assume that the number of electrons is the same as the number of protons.
- Since the number of electrons can tell us the number of protons, we see that atomic number increases as you move left to right in each row.
- The atomic number of the elements shown is now in numerical order.
- The rows of atoms repeat once the valence shell is filled.
- This repetition is why the rows are referred to as periods. There are 7 periods in the Periodic Table (although Figure 4.12 only shows the first three.) The first period contains only hydrogen (atomic number of 1) and helium (atomic number of 2) organized in a single energy shell. single energy shell. The second period contains elements 3 through 10 (lithium through neon) electrons organized into 2 shells. The third period contains elements 11 through 20 (lithium through argon and the electrons are organized into 3 shells.
- This repetition is why the rows are referred to as periods. There are 7 periods in the Periodic Table (although Figure 4.12 only shows the first three.) The first period contains only hydrogen (atomic number of 1) and helium (atomic number of 2) organized in a single energy shell. single energy shell. The second period contains elements 3 through 10 (lithium through neon) electrons organized into 2 shells. The third period contains elements 11 through 20 (lithium through argon and the electrons are organized into 3 shells.
- The number of electrons in the valence shell of each element increases by 1 as you move left to right across the table.
- Because only a certain number of electrons can fit within each shell, as displayed in Figure 4.10, the column number gives us the number of valence electrons for each element. This unlocks the "cheat code" built into the Periodic Table. The number of valence electrons is critical to determining the number of chemical bonds that an element can form so this is invaluable information. And you no longer need to draw a Bohr diagram to determine the number of valence electrons!
- If we compare the Periodic Tables in Figures 4.5 and 4.10, we can see columns 3 through 12 have been hidden from view. to use the "cheat code," focus on columns 1, 2, and 13 through 18.
Did I Get This? 4.2
Using the information provided in the section on subatomic particles, explain how one can use the Periodic Table to determine the numbers of each of the subatomic particles in an atom.
- The number of positively charged protons is equal to the atomic number shown for an element and does not change during chemical reactions.
- We know that the Periodic Table shows electrically neutral atoms, and this means that the number of negatively charged electrons is the same as the number of protons, i.e. the atomic number.
- The mass number of an element is the same as the number of protons plus the number of neutrons. This means we can take the average atomic mass, round it to the nearest whole number, and then subtract the atomic number. This gives us the number of neutrons.
Learn By Doing 4.6
Find sodium on the Periodic Table.
- What is the atomic number of sodium (Na)? 11
- What is the atomic mass of sodium? (Round to the nearest whole number.)
23 amu - How many protons does sodium have?
11 protons - How many neutrons does sodium usually have? 12
The actual atomic mass on the periodic table for Sodium is 22.99, not 23. Why?
- Sodium actually has 11.99 neutrons.
No, atoms cannot have fractions of neutrons. The atomic mass is the average of all sodium atoms. - The actual atomic mass depends on the number of protons and that can be different in different atoms of the same element.
No, the number of protons never varies for an element. Sodium always has 11 protons. - Different atoms of sodium can have different numbers of neutrons. 22.99 is an average value.
Yes, most sodium atoms have 12 neutrons, but there are various isotopes of sodium.
Examine the image below.
Which diagram represents Hydrogen with a mass number of 2? Drawing b shows hydrogen-2.
Explain how you know. A single proton has a mass of 1 and a single neutron has a mass of 1. Drawing b has a single + to represent a proton and a single n to represent a neutron. 1 + 1 = 2 for atom b's mass number. (Electron mass is not considered because it is so very small.)
Learn By Doing 4.7
Look up lithium (Li) on the periodic table. How would the electrons in Li fill the shells of the Li atom?
- Three electrons in the first shell
- One electron in the first shell and two electrons in the second shell
- All three electrons in the second shell
- Two electrons in the first shell and one electron in the second shell
If lithium gives up its third electron, the first shell of the atom is now the outer shell. This outer shell is full. What is the charge of this atom?
- +2
- +1
- -1
- -2
Look up magnesium (Mg) on the periodic table. Determine the number of electrons in the first, second, and third shells of a magnesium atom.
- 1st - 2, 2nd - 9, 3rd - 1
- 1st - 2, 2nd - 8, 3rd - 1
- 1st - 2, 2nd - 9, 3rd - 2
- 1st - 2, 2nd - 8, 3rd - 2
Mg gives up the two electrons in its third shell. This makes the second shell (which is full) the outer shell. What is the charge of Mg?
- +2
- +1
- -1
- -2
Did I Get This? 4.3
Imagine you have a magic wand that can change the number of any subatomic particle.
Describe how changing the number of each of the subatomic particles would alter an atom.
- Changing the number of protons would change the atom into a completely different element. For example, adding a proton to a hydrogen nucleus would transform the atom into helium. It's important to remember that changing the number of protons requires a nuclear reaction, and so does not occur during chemical reactions. This type of nuclear reaction is in fact what our sun does. So much energy is released that we see the light and feel the heat from the site of the reactions, 93 million miles (150 million kilometers) away.
- Changing the number of neutrons would change the atom into a different isotope of the same element. the element would not change since only the number of protons determines the element. The atomic mass would change as well as the isotope. The mass of an atom is determined only by the number of protons and neutrons.
- Changing the number of electrons would transform the atom into a charged particle called an ion. Positively charged ions are called cations and negatively charged ions are called anions.
Learn by Doing 4.8
A molecule would best be described as:
- A stable bond between atoms of at least 2 different elements.
No: Although molecules can contain more than 1 element, many, such as oxygen (O2) contain the same element. - A stable bond between 2 atoms of the same element.
No: Although molecules may be made of more than one atom of the same element (such as O2), many molecules (such as H2O) contain atoms of different elements. - A charged molecule is made up of more than one atom.
No: Molecules are neutral, which aids in their stability. - The first 2 responses are correct.
Yes: Molecules are stable bonds between atoms of the same or differing elements. - All three responses are correct.
No: Molecules are not charged.
Did I Get This? 4.4
Define electronegativity in your own words.
Electronegativity refers to an element's ability to draw electrons toward its nucleus when forming a chemical bond. Elements that have very different electronegativity are more likely to form ionic bonds, those with smaller differences may form polar covalent bonds, and those with similar electronegativities form covalent bonds.
Describe why electronegativity is important when thinking about chemical bonding.
Electronegativity is a way to understand what types of chemical bonds may form between atoms of different elements.
Learn By Doing 4.9
An ionic chemical bond is one in which:
- electrons are removed from one atom and transferred to another atom so that the two atoms become oppositely charged.
Yes: This describes an ionic bond. - outer shell electrons are shared by two atoms so as to satisfactorily fill the outer electron shells of both.
No: This describes a covalent bond. - protons or neutrons are shared by two atoms so as to satisfy the requirements of both.
No: Atoms only share their electrons. - outer shell electrons of one atom are transferred to the inner electron shells of another atom.
No: Atoms interact only through their outer shells.
Using the Periodic Table above, determine whether or not the pair of atoms listed will form an ionic bond.
- Li and Na
No, both of these atoms have only a single valence electron. - K and Br
Yes, K has 1 valence electron while Br has 7. If K gives up an electron and Br accepts that electron, then K will become a cation and Br an anion. These oppositely charged ions will be held together by electrostatic attraction. This combination will form an ionic bond since K is column 1 and Br is Column 7. K transfers an electron to Br. Br becomes a negative ion and now has a full valence shell. K "has a full shell that is "exposed" by giving up its outer shell electron and becomes a positive ion.
Learn By Doing 4.10
Oxygen has an atomic number of eight. How many electrons are in its outermost shell? 2, 4, 6, or 8?
How many covalent bonds can oxygen form to fill its outer shell? 1, 2, 3, 4, or 6?
How many hydrogen atoms will probably form covalent bonds with oxygen? 1, 2, 3, or 4?
What determines the number of covalent bonds formed by an atom?
- Orbitals found in the atom.
No: Only the outer electron shells are involved in chemical bond formation. - Electrons in each orbital in the atom.
No: Only the outer orbitals are involved. - Electrons in the outer valence shell of the atom.
Yes: An atom attempts to fill its outer valence shell. - Electron shells in the atom.
No: Only the outer valence shell is involved in reactions.
Learn By Doing 4.11
To the right is a diagram of an organic molecule.
- What type of diagram is this? Ball and stick, where the "balls" represent atoms, and every single line represents a pair of shared electrons, i.e. a chemical bond.
- Carbon atoms are represented by black spheres. How many carbon atoms are present? 3
- Hydrogen atoms are represented by pale gray spheres. How many hydrogen atoms are present? 6
- Oxygen atoms are represented by red spheres. How many oxygen atoms are present? 3
- What is the chemical formula for this molecule? H6C3O3
- What do the lines between spheres represent? A single line between spheres represents a single pair of shared electrons, otherwise known as a single covalent bond. Two lines between spheres represent 2 pairs of shared electrons, a double covalent bond.
- Bonus: What is the mass of a single molecule of this substance?
The chemical formula is H6C3O3. If we go to the Periodic Table and look up the masses of carbon, hydrogen, and oxygen atoms, we see that the rounded mass of carbon is 12, the rounded mass of hydrogen is 1, and the rounded mass of oxygen is 16. Now we do a bit of math, combining the masses with the chemical formula as shown below:- (6 hydrogen atoms x 1 amu) +( 3 carbon atoms x 12 amu) + (3 oxygen atoms x 16 amu)
- 6 + 36 + 48 = 90 amu
Learn By Doing 4.12
Describe the similarity between polar and non-polar covalent bonds.
Both polar and non-polar covalent bonds involve the sharing of pairs of electrons between atoms.
Describe the difference between polar and non-polar covalent bonds.
The difference between polar and non-polar covalent bonds is that in polar covalent bonds, electrons in a pair are not shared equally between the 2 atoms. This results in areas of partial charge, where the area that is more electronegative (meaning it has more "pulling power") is partially negative since electrons spend more time orbiting that nucleus. The area where electrons spend less time has a partial positive charge.
Which statement is true about hydrogen bonds?
- Hydrogen bonds attract molecules or parts of molecules to each other.
Yes: Specifically, they attract certain H atoms on one molecule or part of a molecule to specific - All of these answers are correct.
No: Only one of these answers is correct. - Hydrogen bonds are formed between oxygen and hydrogen atoms in a water molecule.
No: The bonds between oxygen and hydrogen in water are covalent bonds. Hydrogen bonds do NOT connect atoms to each other, forming molecules. - Hydrogen bonds connect atoms to each other, forming molecules.
No: This option describes covalent bonds. Hydrogen bonds are different.
Hydrogen bonds form in biological systems between molecules that contain hydrogen and what other molecule(s)?
- oxygen
No: Not quite. This is only partially true. Hydrogen bonds form between molecules that contain oxygen and hydrogen. However, this is not the ONLY answer. - nitrogen
No: Not quite. This is only partially true. Hydrogen bonds can form between molecules that contain nitrogen and hydrogen. However, this is not the ONLY answer. - carbon
No: Incorrect. Carbon and hydrogen EQUALLY share electrons, because carbon and hydrogen have similar electronegativities. - oxygen and nitrogen
Correct. Yes, this is the best answer. Both oxygen and nitrogen have high electronegativities.
Learn By Doing 4.13
As you've learned, there are different kinds of chemical bonds-- ionic, covalent, and hydrogen.
Close your eyes and imagine you are so tiny that you can swim around inside a cell. You see lots of molecules bouncing into one another as they interact with the water molecules inside the cell. You notice that molecules formed with a certain kind of bond seem to be more stable in the aqueous environment of our cells. Which type of bond is it? Why is that type of bond the most stable?
The most stable atoms would be those formed by sharing pairs of electrons, called covalent bonds. These bonds have the highest energy in the watery environment of the cell and so would require the most energy to break. Compounds formed by electrostatic attraction between oppositely charged ions (ionic bonds) dissociate when placed in water. Hydrogen bonds are intermolecular forces; they do not form molecules or compounds.
Did I Get This? 4.5
The partial negative charge at one end of a water molecule is attracted to the partial positive charge of another water molecule. This attraction is called:
- a hydration shell
- a hydrogen bond
- an ionic bond
- a covalent bond
- a hydrophobic bond
Which bonds must be broken for water to vaporize?
- hydrogen bonds
- ionic bonds
- hydrophobic interactions
- nonpolar covalent bonds
- polar covalent bonds
Learn By Doing 4.14
The polar character of water that results in its ability to form extensive hydrogen bonding networks gives rise to several properties of water that are important to sustaining life. The following is a list of a few of these properties. Explain why each of these properties is important to sustaining life.
- Water has high surface tension.
- Ice is less dense than water.
- High heat is required for the vaporization of water (a large amount of heat is required to convert liquid water to a gas).
- Water has a high specific heat capacity (a large amount of heat is required to raise the temperature of one gram of water one degree Celsius).
- Water has a high dielectric constant (a measure of the polarity of the polar covalent bond).
OUR ANSWER
- Water's high surface tension allows it to enter small structures (capillary action), allowing water to be transported in plants.
- If ice were more dense, lakes would freeze from the bottom up, never completely thawing in the summer.
- The high heat required for the vaporization of water allows an organism to cool via the evaporation of sweat. It also prevents the body from losing too much water due to evaporation (sweating) when you run a fever or are in a hot climate.
- Water's high specific heat capacity means that heat produced by biochemical reactions within an organism doesn’t raise the temperature of the organism.
- The polarity of water reduces ionic attraction, allowing salts to dissolve.
How do biologists and chemists define the term "organic"?
The term organic is applied to any compound that has 2 or more covalent bonds between carbon and hydrogen atoms. Complex organic molecules are produced by living things.
Did I Get This? 4.6
Describe dehydration synthesis and hydrolysis in your own words.
- Small subunit molecules called monomers are built up into larger molecules using the process of dehydration synthesis. In this process, a molecule of water is produced along with a larger molecule called a polymer.
- Hydrolysis is the process of breaking polymers into smaller molecules one monomer at a time. A molecule of water is required as a reactant for every monomer in the products.
Did I Get This? 4.7
- Differentiate between the terms salt and electrolyte.
There is no true difference IF the salt is dissolved in water. All salts become electrolytes in water and are capable of conducting an electrical current in a solution. This property is critical to the function of ions in transmitting nerve impulses and prompting muscle contraction. - Describe the meaning of "spheres of hydration."
This term refers to the water molecules arranged around ions in solution. Water is polar, with areas of partial charge, 2 negative on the oxygen end of the molecule, and one area of positive partial charge associated with each hydrogen. Salts dissociate into positively and negatively charged ions. Opposite charges attract... so the areas of partial negative charge on the oxygen in water are attracted to positively charged ions and orient themselves around the cation. Anions are surrounded by the positively charged hydrogen ends of the water molecules.
Sea water has a pH around 8 and lemon juice has a pH of 2. Which has the greatest number of free protons in solution?
- Sea water
- Lemon juice
Hint: Refer to the pH scale in the text. Observe that as pH increases, free proton concentration [H+] decreases. Remember as pH increases the concentration of H+ ions decreases.
The difference in pH between lemon juice and seawater is six orders of magnitude. Written out another way, that represents what difference in proton concentration?
- a six-fold difference
- a difference of 1 x 106
- a difference of 0.06
Hint: Orders of magnitude are 10-fold differences. Refer to the scale and count the orders of magnitude between pH 2 and pH 8.
Did I Get This? 4.8
The pH of a solution is a measure of ________________ in the solution.
- the concentration of electrons
- the molecular weight of ions
- total of all ions
- the concentration of H+ ions
Blueberries grow best in moderately acidic soils. Which of the following would be an appropriate pH of the soil in which blueberries can be expected to grow well?
- 1.2
- 7.0
- 12.0
- 4.8
- 8.3
Learn By Doing 4.16
Complete the following sentence: A buffer is... something that helps a solution resist changes in pH. It is usually a combination of weak acid and weak base.
In your own words, describe why buffers are critical to homeostasis in living things.
Living things are sensitive to small changes in pH; buffers help cells and by extension organisms to resist changes in pH.