Control and Regulation 2
18 Endocrine System
Introduction to the Endocrine System
Maintaining homeostasis within the body requires the coordination of many different systems and organs. Communication between neighboring cells and between cells and tissues in distant parts of the body is done through either the nervous system or the endocrine system. The endocrine system regulates biological processes through the release of chemicals called hormones. Hormones are released into body fluids— usually, blood, which carries these chemicals to their target cells, where they elicit a response. The responses elicited by hormones usually take several seconds to several days to occur, and the duration of the response can last just as long without further signaling. Unlike nearly instantaneous nerve impulses, hormones of the endocrine system can regulate functions of the body on longer time scales to maintain homeostasis.
Learn By Doing 18.1
Decide if the nervous or endocrine system is responsible for each of the following:
- Chemicals transported in the blood
- Quick initiation and conclusion of regulatory effect in the body
- Slow initiation but a long-term regulatory effect on the body
- Directly regulates the metabolism of tissues and cells
Structure and Function of the Endocrine System
Learning Objectives
- Describe the major functions of the endocrine system.
- Identify major diseases associated with the endocrine system.
- Define homeostasis.
The endocrine system consists of cells, tissues, and organs that secrete hormones as a primary or secondary function. The endocrine gland is the major player in this system. The primary function of these ductless glands is to secrete their hormones directly into the surrounding fluid. The interstitial fluid and the blood vessels then transport the hormones throughout the body.
The major function of the endocrine system is to participate in homeostatic feedback loops by acting as a means of communication between integrators and effectors and sometimes acting as the sensor as well. Since the function of the endocrine system is to maintain homeostasis, there will be many system-level examples at the end of this chapter.
The endocrine system is also involved with growth, development, and adaptation. Within all of these changing processes, our bodies tolerate fluctuations within certain limits, but still, overall homeostasis needs to be maintained.
Example: Endocrine System Homeostasis and Integration with Other Systems
When we grow in height, our bones increase in length. However, proper homeostasis must be maintained to ensure that we always have calcified bone to support our weight. Muscles, tendons, and ligaments must also grow proportionately. The endocrine system integrates these changes while still maintaining homeostasis.
We feel the effects of changes in the endocrine system at various points in our lives. Hormone levels fluctuate during puberty and even after we eat a meal. Common dysfunctions of the endocrine system include an inability to regulate glucose, called diabetes mellitus, and an inability to regulate calcium levels in the bones, which may lead to osteoporosis. Other common disorders of the endocrine system include over- or under-production of thyroid hormone (hyperthyroidism and hypothyroidism, respectively), which impacts energy metabolism.
The endocrine system includes the pituitary, thyroid, parathyroid, adrenal, and pineal glands as illustrated in the figure below. Some glands have both endocrine and non-endocrine functions. For example, the pancreas contains cells that function in digestion as well as cells that secrete the hormones insulin and glucagon, which regulate blood glucose levels. The hypothalamus, thymus, female ovaries, and male testes are other organs that contain cells with endocrine functions. Moreover, adipose tissue has long been known to produce hormones, and recent research has revealed that even bone tissue has endocrine functions.
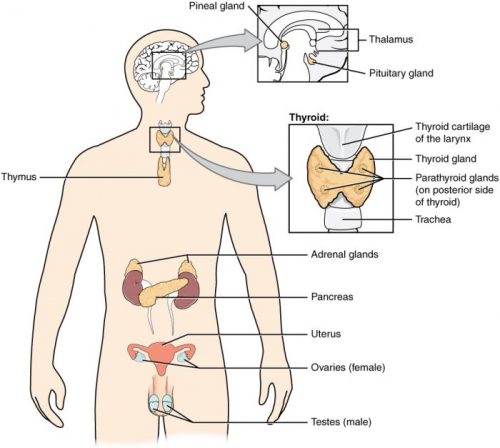
The ductless endocrine glands are not to be confused with the body’s exocrine system, whose glands release their secretions through ducts. Examples of exocrine glands include the sebaceous and sweat glands of the skin. As just noted, the pancreas also has an exocrine function: most of its cells secrete pancreatic juice through the pancreatic and accessory ducts to the lumen of the small intestine.
Learn By Doing 18.2
If you had to summarize the function of the endocrine system in one word, what would it be?
- Communication
- Homeostasis
- Development
- Growth
Which of the following is not a function of the endocrine system?
- Produce quick effects through electrochemical mechanisms.
- Release chemicals into the bloodstream for distribution throughout the body.
- Contribute to homeostatic feedback loops.
- Alter the metabolic activities of many different target tissues and organs.
Hint: The endocrine system will share some characteristics with the other major coordinating system, the nervous system, but it will have some major differences from both the nervous system and local cell- or tissue-level communication.
Endocrine glands ________.
- secrete hormones that travel through a duct to the target organs
- release neurotransmitters into the synaptic cleft
- secrete chemical messengers that travel in the bloodstream
- include sebaceous glands and sweat glands
Endocrine Levels of Organization
We will describe the molecular and organ levels of organization within the endocrine system. Our discussion of hormones and hormone receptors as molecules illustrates the relationship between structure and function.
Hormones
Learning Objectives
- Define hormone.
- Identify different types of hormones.
- Explain the regulation of hormone production.
Hormones are the chemical messengers of the endocrine system. They elicit specific responses by binding to receptors on or in target cells.
The amount of a hormone circulating at any one time is affected by
- the rate of hormone production
- the rate of secretion from endocrine glands
- the rate of blood flow delivering the hormone to its target cells with specific receptors
- the availability and number of hormone receptors
- the rate of biochemical degradation and elimination of the hormone
The time it takes the body to degrade 50 percent of a predetermined amount of hormone is called the hormone’s half-life. A hormone with a long half-life would persist in the blood long after the endocrine gland had stopped releasing it.
Many of these factors are a function of the type of hormone produced. Although there are many different hormones in the human body, they can be divided into three classes based on their chemical structure: lipid-derived, amino acid-derived, and peptide (protein) hormones. One of the key distinguishing features of lipid-derived hormones is that they can diffuse across plasma membranes whereas amino acid-derived and peptide hormones cannot.
Lipid-Derived Hormones
Most lipid hormones are derived from cholesterol and thus are structurally similar to it, as illustrated below. The primary class of lipid hormones in humans is steroid hormones. Examples include estradiol, which is an estrogen, or female sex hormone, and testosterone, which is an androgen, or male sex hormone. These two hormones are released by the female and male reproductive organs, respectively. Other steroid hormones include aldosterone and cortisol, which are released by the adrenal glands along with some other types of androgens. Steroid hormones are insoluble in water, so they are transported by transport proteins in the blood. As a result, they remain in circulation longer than peptide hormones. For example, cortisol has a half-life of 60 to 90 minutes, while epinephrine, an amino acid derived-hormone, has a half-life of approximately one minute.
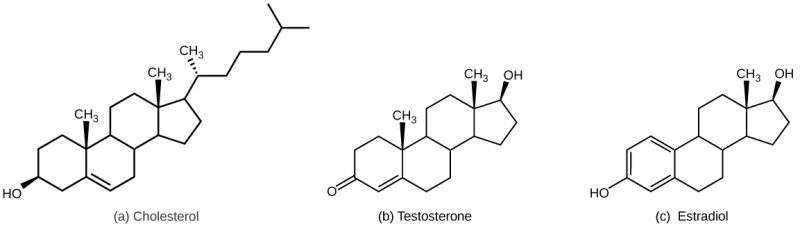
Amino Acid-Derived Hormones
The amino acid-derived hormones are relatively small molecules that are derived from the amino acids tyrosine and tryptophan, shown below. If a hormone is amino acid-derived, its chemical name will end in –ine. Examples of amino acid-derived hormones include epinephrine and norepinephrine, which are synthesized in the medulla of the adrenal glands, and thyroxine, which is produced by the thyroid gland. The pineal gland in the brain makes and secretes melatonin which regulates sleep cycles.
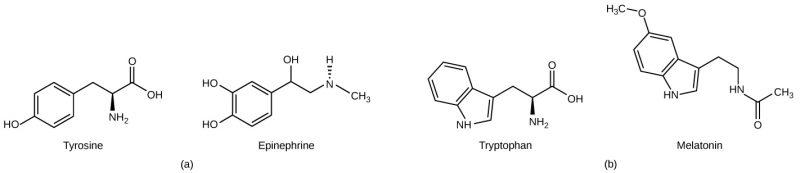
Peptide Hormones
The structure of peptide hormones is that of a polypeptide chain (chain of amino acids). The peptide hormones include molecules that are short polypeptide chains, such as antidiuretic hormone and oxytocin produced in the brain and released into the blood in the posterior pituitary gland. This class also includes small proteins, like growth hormones produced by the pituitary, and large glycoproteins such as follicle-stimulating hormone produced by the pituitary. The figure below illustrates a number of peptide hormones.
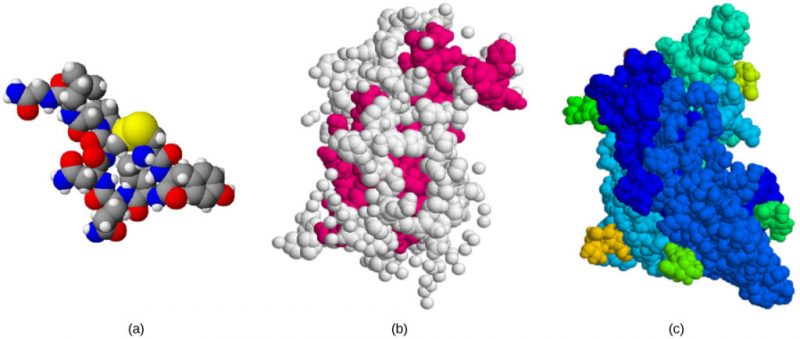
Secreted peptides like insulin are stored within vesicles in the cells that synthesize them. They are then released in response to stimuli such as high blood glucose levels in the case of insulin. Amino acid-derived and polypeptide hormones are water-soluble and insoluble in lipids. These hormones cannot pass through the plasma membranes of cells; therefore, their receptors are found on the surface of the target cells.
Hormone Receptors
Hormones mediate changes in cells by binding to specific receptors; individual hormones have their own unique receptor or class of receptors. A cell that has a receptor for a specific hormone is called a target cell. A hormone can therefore circulate throughout the body, contacting many different cell types but affecting only those cells that possess the specific receptor. A hormone receptor is a protein (cell membrane or intracellular) that binds specifically to a particular hormone. Receptors for a specific hormone may be found in or on many different target cells or may be limited to a small number of specialized cells.
Hormones bind to receptors and cause changes in cellular function. Receptors can be found either inside the cell (intracellular receptors) or on the cell’s surface (plasma membrane receptors). Knowing the location of a specific receptor provides important information about the mechanism that specific hormone uses to affect cell physiology.
Learn By Doing 18.3
Which of the following accurately describes hormones?
- Circulate indefinitely in the body
- Are chemical messengers
- Are neural messengers
- Elicit a body-wide response from all cell types
A cell that has a receptor for a specific hormone is called a:
- Target cell
- Specialized cell
- Differentiated cell
- Endocrine cell
What would happen to a body cell that doesn’t have a specific receptor for a specific hormone that is released and circulating in the blood?
- The cell would decrease its physiological response to that hormone.
- The cell would not respond to the hormone.
- The cell would increase its physiological response to that hormone
What would happen to a body cell if another chemical, such as a drug, completely blocked a specific hormone receptor?
- The cell would die.
- The cell would not respond to the hormone.
- The cell would increase its physiological response to that hormone.
How Hormones Work
Learning Objectives
- Describe the two types of hormone receptors.
- Describe the mechanism of action of the two major types of hormones.
Hormones cause cellular changes by binding to receptors on target cells. The number of receptors on a target cell can increase or decrease in response to hormone activity. Hormones can affect cells directly through intracellular hormone receptors or indirectly through plasma membrane hormone receptors.
Hormones mediate changes in target cells by binding to specific hormone receptors. In this way, even though hormones circulate throughout the body and come into contact with many different cell types, they only affect cells that possess the necessary receptors. Receptors for a specific hormone may be found on many different cells or may be limited to a small number of specialized cells. For example, thyroid hormones act on many different tissue types, stimulating metabolic activity throughout the body. Cells can have many receptors for the same hormone but often also possess receptors for different types of hormones.
The number of receptors that respond to a hormone determines the cell’s sensitivity to that hormone and the resulting cellular response. Additionally, the number of receptors that respond to a hormone can change over time, resulting in increased or decreased cell sensitivity. In up-regulation, the number of receptors increases in response to rising hormone levels, making the cell more sensitive to the hormone and allowing for more cellular activity. When the number of receptors decreases in response to rising hormone levels, called down-regulation, cellular activity is reduced.
Receptor binding alters cellular activity and results in an increase or decrease in normal body processes. Depending on the location of the protein receptor on the target cell and the chemical structure of the hormone, hormones can mediate changes directly by binding to intracellular hormone receptors and modulating gene transcription or indirectly by binding to cell surface receptors and stimulating signaling pathways.
Intracellular Hormone Receptors
Steroid hormones are lipophilic and need to be transported by proteins in the blood. Once released from their transport protein, the non-polar hormone is able to diffuse across the plasma membrane of cells. Recall that the lipid bilayer of the plasma membrane of cells uses amphiphilic phospholipids to compartmentalize the cytoplasm of a cell. When a steroid hormone crosses the plasma membrane of a target cell, it binds to an intracellular hormone receptor in the cytoplasm, on an intracellular membrane system (ER), or within the nucleus of the cell. The receptor/hormone complex can then bind to a specific site on DNA and act as a transcription regulator to increase or decrease the synthesis of particular mRNA molecules coded by these specific genes. This, in turn, alters mRNA production, which determines the amount of corresponding protein that is synthesized. The steroid hormone regulates specific cell processes. The rate of transcription and protein synthesis is directly proportional to the amount of hormone-forming receptor/hormone complexes; so, if the hormone production increases, so do the physiological effect in the body.
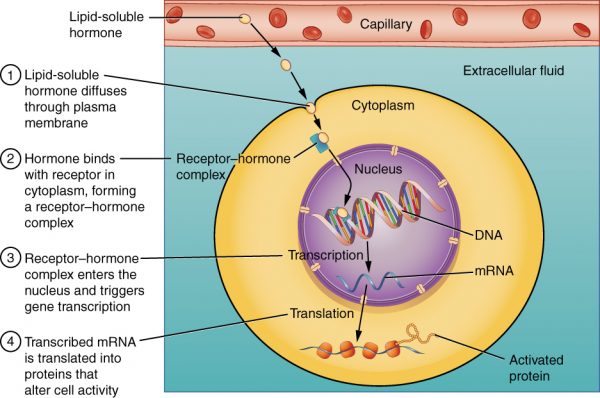
The cell signaling pathways induced by the steroid hormones regulate specific genes on the cell’s DNA. The hormones and receptor complex act as transcription regulators by increasing or decreasing the synthesis of mRNA molecules of specific genes. This, in turn, determines the amount of corresponding protein that is synthesized by altering gene expression. This protein can be used either to change the structure of the cell or to produce enzymes that catalyze chemical reactions. In this way, the steroid hormone regulates specific cell processes.
Other lipid-soluble hormones that are not steroid hormones, such as vitamin D and thyroxine, have receptors located in the nucleus. The hormones diffuse across both the plasma membrane and the nuclear envelope, then bind to receptors in the nucleus. The hormone-receptor complex stimulates the transcription of specific genes.
Plasma Membrane Hormone Receptors
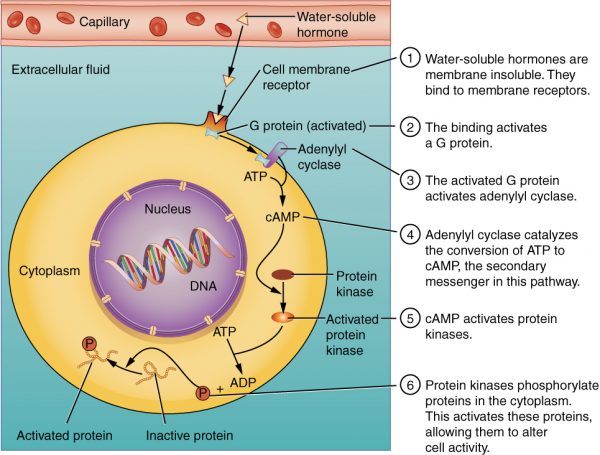
Amino acid-derived hormones and polypeptide hormones are not lipid-derived (lipid-soluble) and, therefore cannot diffuse through the plasma membrane of cells. Lipid-insoluble hormones bind to receptors on the outer surface of the plasma membrane via plasma membrane hormone receptors. Unlike steroid hormones, lipid-insoluble hormones do not directly affect the target cell because they cannot enter the cell and act directly on DNA. Binding of these hormones to a cell surface receptor results in the activation of a signaling pathway; this triggers intracellular activity and carries out the specific effects associated with the hormone. In this way, nothing passes through the cell membrane; the hormone that binds at the surface remains at the surface of the cell while the intracellular product remains inside the cell. The hormone that initiates the signaling pathway is called a first messenger, which activates a second messenger in the cytoplasm.
One very important second messenger is cyclic AMP (cAMP). When a hormone binds to its membrane receptor, a G-protein that is associated with the receptor is activated; G-proteins are proteins separate from receptors that are found in the cell membrane. When a hormone is not bound to the receptor, the G-protein is inactive and is bound to guanosine diphosphate or GDP. When a hormone binds to the receptor, the G-protein is activated by binding guanosine triphosphate, or GTP, in place of GDP. After binding, GTP is hydrolyzed by the G-protein into GDP and becomes inactive.
The activated G-protein in turn activates a membrane-bound enzyme called adenyl cyclase. Adenyl cyclase catalyzes the conversion of ATP to cAMP. cAMP, in turn, activates a group of proteins called protein kinases, which transfer a phosphate group from ATP to a substrate molecule in a process called phosphorylation. The phosphorylation of a substrate molecule changes its structural orientation, thereby activating it. These activated molecules can then mediate changes in cellular processes.
The effect of a hormone is amplified as the signaling pathway progresses. The binding of a hormone at a single receptor causes the activation of many G-proteins, which activates adenylyl cyclase. Each molecule of adenyl cyclase then triggers the formation of many molecules of cAMP. Further amplification occurs as protein kinases, once activated by cAMP, can catalyze many reactions. In this way, a small amount of hormone can trigger the formation of a large amount of cellular product. To stop hormone activity, cAMP is deactivated by the cytoplasmic enzyme phosphodiesterase or PDE. PDE is always present in the cell and breaks down cAMP to control hormone activity, preventing the overproduction of cellular products.
The specific response of a cell to a lipid-insoluble hormone depends on the type of receptors that are present on the cell membrane and the substrate molecules present in the cell cytoplasm. Cellular responses to hormone binding of a receptor include altering membrane permeability and metabolic pathways, stimulating the synthesis of proteins and enzymes, and activating hormone release.
Learn By Doing 18.4
Which of the following is correctly matched?
- Steroid hormones bind to a nuclear receptor. Thyroid hormones bind to a cytoplasmic receptor.
- Steroid hormones bind to a cytoplasmic receptor. Thyroid hormones bind to a nuclear receptor.
- Steroid hormones bind to an intracellular receptor. Thyroid hormones bind to a plasma membrane receptor.
- Steroid hormones bind to a plasma membrane receptor. Thyroid hormones bind to an intracellular receptor.
Peptide hormones must bind to a plasma membrane hormone receptor because:
- They are too big.
- They are nonpolar.
- They are polar.
- They are small.
A newly developed pesticide has been observed to bind to an intracellular hormone receptor. If ingested, residue from this pesticide could disrupt the effects of ________.
- melatonin
- estrogen
- growth hormone
- insulin
A small molecule binds to a G protein, preventing its activation. What direct effect will this have on signaling that involves cAMP?
- The hormone will not be able to bind to the hormone receptor.
- Adenyl cyclase will not be activated.
- Excessive quantities of cAMP will be produced.
- The phosphorylation cascade will be initiated.
Why is it important to know the location of a hormone receptor?
- It is important because it provides information about how the hormone will affect the cell.
- It is important because it tells exactly what hormone it will bind.
- It is important because it tells what type of hormone will be produced.
- It is not important because all receptors will work the same way.
Hormone Regulation
Learning Objective
Positive and negative feedback pathways can regulate hormone production. In positive feedback systems, the release of a hormone leads to an action that stimulates the release of more of the same hormone.
Example: Positive Feedback Loop in the Endocrine System
Oxytocin released by the pituitary gland prior to childbirth stimulates the contraction of the uterus and increases pressure on the cervix. The increased pressure signals the pituitary to release even more oxytocin, which increases the force of contractions, leading to even more cervical pressure. This amplification cycle continues until childbirth is complete.
In a hormonal negative feedback loop, when a stimulus causes the release of a hormone (Hormone A), the hormone binds to the target cell receptor, causing the necessary metabolic change toward homeostasis. In a negative loop, the effect of this metabolic change is to counter the stimulus that caused the release of Hormone A. Once the cause of the stimulus returns to the normal range, the production of that hormone stops and the plasma level of that hormone returns to the normal (pre-stimulus) level. In this way, the concentration of most hormones in the blood is maintained within a narrow range.
Example: Negative Feedback Loop in the Endocrine System
The hypothalamus monitors the plasma level of thyroid hormones, among others. When the level drops, the hypothalamus stimulates the anterior pituitary to release a hormone (Thyroid Stimulating Hormone, or Thyrotropin) that stimulates the thyroid to release thyroid hormones. Increased levels of the thyroid hormones in the blood then feedback to the hypothalamus and anterior pituitary to inhibit further stimulation of the thyroid gland. Other homeostatic imbalances, such as low body temperature, can also stimulate the hypothalamus to stimulate the anterior pituitary to release thyrotropin. Thyroid hormones play an important role in metabolic heat production via ATP production.
Learn By Doing 18.5
Using this diagram to illustrate the childbirth example, which letter on this positive feedback loop image would represent the release of the hormone oxytocin?
Hint: The hormone is the chemical message that is being released in response to the stimulus; other communication would be neural between the sensor and control center.
For oxytocin, what type of receptor would be involved in this positive feedback loop?
- chemoreceptor
- thermoreceptor
- mechanoreceptor
- photoreceptor
Hint: The receptors are located in the cervix and uterus.
For oxytocin, what would be the control center?
- cervix
- mammary gland
- pancreas
- pituitary
Hint: This control center is releasing the hormone oxytocin when stimulated by the input from the receptor.
The cervical and uterine tissue becomes more sensitive to oxytocin during late pregnancy in response to increased levels of estrogen in the mother’s blood. What might be changing to make this tissue more sensitive to oxytocin and enhance this positive feedback?
A positive feedback loop is unstable and would result in a runaway situation if something did not terminate the continued increased stimulus. In the example of childbirth, why does the feedback loop stop eventually?
The contractions will continue for minutes to an hour after the baby is born. This helps expel the placenta, also known as afterbirth. Why would the contractions continue for this time period even though the baby has been born?
Learn By Doing 18.6
This diagram illustrates the negative feedback mechanism involving three hormones.
Which letter on this negative feedback loop image would represent the release of the hormone that is the “opposite change” restoring homeostasis?
Hint: The gland producing the hormone being monitored would be the final “effector” in this loop.
In this negative feedback loop, there are two “control centers” receiving feedback from the change initiated by the effector. One is neural, and one is endocrine. A similar control relationship between these two exists for several tropic hormones that control other endocrine glands. Based on this, which endocrine gland is sometimes called the “master gland”?
- anterior pituitary gland
- posterior pituitary gland
- hypothalamus
- thyroid gland
Hint: In this scenario, the hypothalamus would be the “master” of the “master gland”.
Look at the letter “E” on the feedback diagram. What would be the advantage of input from other parts of the brain continuing to stimulate the hypothalamus, and thus more thyroid hormone production even if the blood level has returned within the normal homeostatic range?
Hint: Most body cells have receptors for thyroid hormone and it stimulates increased metabolism. A by-product of cell metabolism is heat. People with an underactive thyroid gland are at higher risk for hypothermia.
Elevated levels of cortisol, also known as the “stress hormone”, can inhibit the production of thyroid-stimulating hormone from the anterior pituitary. What would be the effect on the production of thyroid hormone by the thyroid gland?
- increased production of thyroid hormone
- decreased production of thyroid hormone
- stable production of thyroid hormone
Hint: Chronic stress is often related to symptoms of hypothyroidism.
The autoimmune condition Grave’s disease results in antibodies in the blood that bind to thyroid-stimulating hormone receptors, mimicking their metabolic effect on the target cell. What would be the effect on the production of thyroid hormone by the thyroid gland?
- increased production of thyroid hormone
- decreased production of thyroid hormone
- stable production of thyroid hormone
Hint: Grave’s disease often causes symptoms of hyperthyroidism.
A different autoimmune Hashimoto’s thyroiditis disease results in the destruction of thyroid cells by lymphocytes. What would be the effect on the production of thyroid hormone by the thyroid gland?
- increased production of thyroid hormone
- decreased production of thyroid hormone
- stable production of thyroid hormone
Hint: A person with Hashimoto’s thyroiditis exhibits symptoms of hypothyroidism.
What would be the advantage of having the negative feedback from the increased thyroid hormones to both the hypothalamus and the anterior pituitary?
Hint: Consider what would happen if only the hypothalamus received feedback. Think about one of the major differences between neural and endocrine control.
Did I Get This? 18.1
Which method of hormone production is going to help return the body to homeostasis?
- Positive Feedback
- Negative Feedback
Homeostatic mechanisms stimulated by changes in body fluid composition (humoral) often involve more than one hormone in a negative feedback loop. Why would this be typical? For example, insulin decreases blood glucose while glucagon increases blood glucose; parathyroid hormone increases blood calcium levels while calcitonin from the thyroid decreases blood calcium in children.
Stimuli that Regulate Hormone Production
Learning Objective
During hormone regulation, hormones are released either directly by an endocrine gland or indirectly through the action of the hypothalamus of the brain, which stimulates other endocrine glands to release hormones in order to maintain homeostasis. The hormones activate target cells, which initiate physiological changes that adjust the body’s conditions. When normal conditions have been recovered, the corrective action – the production of hormones – is discontinued. Thus, in negative feedback, when the original (abnormal) condition has been repaired or negated, corrective actions decrease or discontinue.
In another example of hormone regulation, the anterior pituitary signals the thyroid to release thyroid hormones. Increasing levels of these hormones in the blood then give feedback to the hypothalamus and anterior pituitary to inhibit further signaling to the thyroid gland, as illustrated below.
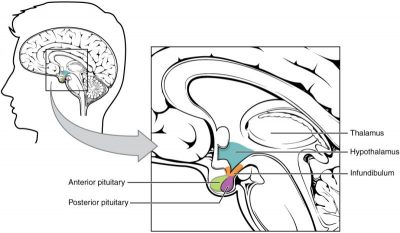
There are three mechanisms by which endocrine glands are stimulated to synthesize and release hormones: humoral stimuli, hormonal stimuli, and neural stimuli.
The term “humoral” is derived from the term “humor,” which refers to bodily fluids such as blood. The term “humoral stimuli” refers to the control of hormone release in response to changes in extracellular fluids such as blood or the ion concentration in the blood. For example, a rise in blood glucose levels triggers the pancreatic release of insulin. Insulin causes blood glucose levels to drop, which signals the pancreas to stop producing insulin in a negative feedback loop.
The term “hormonal stimuli” refers to the release of a hormone in response to another hormone. A number of endocrine glands release hormones when stimulated by hormones released by other endocrine glands. For example, the hypothalamus produces hormones that stimulate the anterior portion of the pituitary gland. The anterior pituitary in turn releases hormones that regulate hormone production by other endocrine glands. The anterior pituitary releases the thyroid-stimulating hormone, which then stimulates the thyroid gland to produce the hormones T3 and T4. As blood concentrations of T3 and T4 rise, they inhibit both the pituitary and the hypothalamus in a negative feedback loop. Sometimes students get confused between tropic and trophic hormones. Tropic hormones stimulate the release of other hormones from endocrine cells, such as those discussed here.Trophic (meaning “nourishment or nurse”) hormones stimulate non-endocrine cell growth and development, such as growth hormone, estrogen, and testosterone.
In some cases, the nervous system directly stimulates endocrine glands to release hormones, something referred to as neural stimuli. For example, in a short-term stress response, the hormones epinephrine and norepinephrine are important for providing the bursts of energy required for the body to respond. In this case, neuronal signaling from the sympathetic nervous system directly stimulates the adrenal medulla to release the hormones epinephrine and norepinephrine in response to stress.
Learn By Doing 18.7
Hormones that control the release of another hormone are called
- Trophic
- Tropic
- Humor
- Triggers
Endocrine Glands
Learning Objective
- Describe the role of the hypothalamic-pituitary axis in the endocrine system
- Describe the role of the thyroid and parathyroid glands in the endocrine system
- Describe the role of the adrenal gland in the endocrine system
- Describe the role of the pancreas, pineal gland, and gonads in the endocrine system
- Identify different organs that have secondary endocrine functions
Both the endocrine and nervous systems use chemical signals to communicate and regulate the body’s physiology. The endocrine system releases hormones that act on target cells to regulate development, growth, energy metabolism, reproduction, and many behaviors. The nervous system releases neurotransmitters or neurohormones that regulate neurons, muscle cells, and endocrine cells. Because the neurons can regulate the release of hormones, the nervous and endocrine systems work in a coordinated manner to regulate the body’s physiology.
Hypothalamic-Pituitary Axis
The hypothalamus in vertebrates integrates the endocrine and nervous systems. The hypothalamus is an endocrine organ located in the diencephalon of the brain. It receives input from the body and other brain areas and initiates endocrine responses to environmental changes. The hypothalamus acts as an endocrine organ, synthesizing hormones and transporting them along axons to the posterior pituitary gland. It synthesizes and secretes regulatory hormones that control the endocrine cells in the anterior pituitary gland. The hypothalamus contains autonomic centers that control endocrine cells in the adrenal medulla via neuronal control.
The pituitary gland, sometimes called the hypophysis or “master gland” is located at the base of the brain in the sella turcica, a groove of the sphenoid bone of the skull, illustrated in the figure above. It is attached to the hypothalamus via a stalk called the pituitary stalk(or infundibulum). The anterior portion of the pituitary gland is regulated by releasing or release-inhibiting hormones produced by the hypothalamus, and the posterior pituitary receives signals via neurosecretory cells to release hormones produced by the hypothalamus. The pituitary has two distinct regions—the anterior pituitary and the posterior pituitary—which between them secrete nine different peptide or protein hormones. The posterior lobe of the pituitary gland contains axons of the hypothalamic neurons.
Anterior Pituitary
The anterior pituitary gland, or adenohypophysis, is surrounded by a capillary network that extends from the hypothalamus, down along the infundibulum, and to the anterior pituitary. This capillary network is a part of the hypophyseal portal system that carries substances from the hypothalamus to the anterior pituitary and hormones from the anterior pituitary into the circulatory system. A portal system carries blood from one capillary network to another; therefore, the hypophyseal portal system allows hormones produced by the hypothalamus to be carried directly to the anterior pituitary without first entering the circulatory system.
The anterior pituitary produces seven hormones: growth hormone (GH), prolactin (PRL), thyroid-stimulating hormone (TSH), melanin-stimulating hormone (MSH), adrenocorticotropic hormone (ACTH), follicle-stimulating hormone (FSH), and luteinizing hormone (LH). Anterior pituitary hormones are sometimes referred to as tropic hormones because they control the functioning of other organs. While the anterior pituitary produces these hormones, their production is controlled by regulatory hormones produced by the hypothalamus. These regulatory hormones can be releasing hormones or inhibiting hormones, causing more or less of the anterior pituitary hormones to be secreted. These travel from the hypothalamus through the hypophyseal portal system to the anterior pituitary where they exert their effect. Negative feedback then regulates how much of these regulatory hormones are released and how much anterior pituitary hormone is secreted.
Posterior Pituitary
The posterior pituitary is significantly different in structure from the anterior pituitary. It is a part of the brain extending down from the hypothalamus and contains mostly nerve fibers and glial cells, which support axons that extend from the hypothalamus to the posterior pituitary. The posterior pituitary and the infundibulum together are referred to as the neurohypophysis.
The hormones antidiuretic hormone (ADH), also known as vasopressin and oxytocin are produced by neurons in the hypothalamus and transported within these axons along the infundibulum to the posterior pituitary. They are released into the circulatory system via neural signaling from the hypothalamus. ADH and oxytocin are considered to be posterior pituitary hormones because that is where they are released into the circulatory system. The posterior pituitary itself does not produce hormones, but instead stores hormones produced by the hypothalamus and releases them into the bloodstream.
Thyroid Gland
The thyroid gland is located in the neck, just below the larynx, and in front of the trachea. It is a butterfly-shaped gland with two lobes that are connected by the isthmus. It has a dark red color due to its extensive vascular system. When the thyroid swells due to dysfunction, it can be felt under the skin of the neck.
The thyroid gland is made up of many spherical thyroid follicles, which are lined with a simple cuboidal epithelium. These follicles contain a viscous fluid called colloid, which stores the glycoprotein thyroglobulin, the precursor to the thyroid hormones. The follicles produce hormones that can be stored in the colloid or released into the surrounding capillary network for transport to the rest of the body via the circulatory system.
Thyroid follicle cells synthesize the hormone thyroxine, which is also known as T4 because it contains four atoms of iodine, and triiodothyronine, also known as T3 because it contains three atoms of iodine. Follicle cells are stimulated to release stored T3 and T4 by thyroid stimulating hormone (TSH), which is produced by the anterior pituitary. These thyroid hormones increase the rates of mitochondrial ATP production.
A third hormone, calcitonin, is produced by parafollicular cells of the thyroid. TSH does not control release Calcitonin release is not controlled by TSH. Instead, calcitonin is released when calcium ion concentrations in the blood rise. Calcitonin helps to regulate calcium concentrations in body fluids.
Parathyroid Glands
Most people have four parathyroid glands; however, the number can vary from two to six. These glands are located on the posterior surface of the thyroid gland. Normally, there is a superior gland and an inferior gland associated with each of the thyroid’s two lobes. Each parathyroid gland is covered by connective tissue and contains many secretory cells that are associated with a capillary network.
The parathyroid glands produce parathyroid hormone (PTH). PTH increases blood calcium concentrations when calcium ion levels fall below normal.PTH and calcitonin work in opposition to one another to maintain homeostatic Ca2+ levels in body fluids. These hormones encourage bone growth, muscle mass, and blood cell formation in children and women.PTH is produced by the chief cells of the parathyroid.
Adrenal Glands
The adrenal glands are associated with the kidneys; one gland is located on top of each kidney. The adrenal glands consist of an outer adrenal cortex and an inner adrenal medulla. These regions secrete different hormones.
The adrenal cortex is made up of layers of epithelial cells and associated capillary networks. These layers form three distinct regions: an outer zona glomerulosa that produces mineralocorticoids, a middle zona fasciculata that produces glucocorticoids, and an inner zona reticularis that produces androgens. The main mineralocorticoid is aldosterone, which regulates the concentration of Na+ ions in urine, sweat, pancreas, and saliva. Aldosterone release from the adrenal cortex is stimulated by a decrease in blood concentrations of sodium ions, blood volume, or blood pressure or by an increase in blood potassium levels.
The three main glucocorticoids are cortisol, corticosterone, and cortisone. The glucocorticoids stimulate the synthesis of glucose and gluconeogenesis (converting a non-carbohydrate to glucose) by liver cells, and they promote the release of fatty acids from adipose tissue. These hormones increase blood glucose levels to maintain levels within a normal range between meals. These hormones are secreted in response to ACTH, and levels are regulated by negative feedback.
Androgens are sex hormones that promote masculinity. They are produced in small amounts by the adrenal cortex in both males and females. They do not affect sexual characteristics and may supplement sex hormones released from the gonads.
The adrenal medulla contains large, irregularly shaped cells that are closely associated with blood vessels. Preganglionic autonomic nerve fibers from the central nervous system innervate these cells.
The adrenal medulla contains two types of secretory cells: one that produces epinephrine (adrenaline) and another that produces norepinephrine (noradrenaline). Epinephrine is the primary adrenal medulla hormone accounting for 75 to 80 percent of its secretions. Epinephrine and norepinephrine increase heart rate, breathing rate, cardiac muscle contractions, blood pressure, and blood glucose levels. They also accelerate the breakdown of glucose in skeletal muscles and stored fats in adipose tissue.
Neural impulses from the sympathetic nervous system stimulate the release of epinephrine and norepinephrine. Secretion of these hormones is stimulated by acetylcholine release from preganglionic sympathetic fibers innervating the adrenal medulla. These neural impulses originate from the hypothalamus in response to stress to prepare the body for the fight-or-flight response.
Pancreas
The pancreas is an elongated organ that is located between the stomach and the proximal portion of the small intestine. It contains both exocrine cells that excrete digestive enzymes and endocrine cells that release hormones. It is sometimes referred to as a heterocrine gland because it has both endocrine and exocrine functions.
The endocrine cells of the pancreas form clusters called pancreatic islets or the islets of Langerhans. The pancreatic islets contain two primary cell types: alpha cells, which produce the hormone glucagon, and beta cells, which produce the hormone insulin. These hormones regulate blood glucose levels. As blood glucose levels decline, alpha cells release glucagon to raise the blood glucose levels by increasing rates of glycogen breakdown and glucose release by the liver. When blood glucose levels rise, such as after a meal, beta cells release insulin to lower blood glucose levels by increasing the rate of glucose uptake in most body cells and by increasing glycogen synthesis in skeletal muscles and the liver. Together, glucagon and insulin regulate blood glucose levels.
Pineal Gland
The pineal gland produces melatonin. The rate of melatonin production is affected by the photoperiod. Collaterals from the visual pathways innervate the pineal gland. During the day photoperiod, little melatonin is produced; however, melatonin production increases during the dark photoperiod (night). In some mammals, melatonin has an inhibitory effect on reproductive functions by decreasing the production and maturation of sperm, oocytes, and reproductive organs. Melatonin is an effective antioxidant, protecting the CNS from free radicals such as nitric oxide and hydrogen peroxide. Lastly, melatonin is involved in biological rhythms, particularly circadian rhythms such as the sleep-wake cycle and eating habits.
Gonads
The gonads—the male testes and female ovaries—produce steroid hormones. The testes produce androgens, testosterone being the most prominent, which allow for the development of secondary sex characteristics and the production of sperm cells. The ovaries produce estradiol and progesterone, which cause secondary sex characteristics and prepare the body for childbirth.
Endocrine Gland | Associated Hormones | Effect |
Hypothalamus | ||
releasing and inhibiting hormones | regulate hormone release from the pituitary gland | |
oxytocin | produce uterine contractions and milk secretion in females | |
antidiuretic hormone (ADH) | water reabsorption from kidneys; vasoconstriction to increase blood pressure | |
Pituitary (Anterior) | ||
prolactin (PRL) | promotes milk production | |
growth hormone (GH) | promotes the growth of body tissues, protein synthesis; metabolic functions | |
thyroid stimulating hormone (TSH) | stimulates thyroid hormone release | |
adrenocorticotropic hormone (ACTH) | stimulates glucocorticoid hormone release by the adrenal cortex | |
follicle-stimulating hormone (FSH) | stimulates gamete production (both ova and sperm); secretion of estradiol | |
luteinizing hormone (LH) | stimulates androgen production by gonads; ovulation, secretion of progesterone | |
melanocyte-stimulating hormone (MSH) | stimulates melanocytes of the skin increasing melanin pigment production. | |
Thyroid | thyroxine, triiodothyronine | stimulate and maintain metabolism; growth and development |
calcitonin | reduces blood Ca2+ levels | |
Parathyroid | parathyroid hormone (PTH) | increases blood Ca2+ levels |
Adrenal (Cortex) | aldosterone | increases blood Na+ levels; increase K+ secretion |
cortisol, corticosterone, cortisone | increase blood glucose levels; anti-inflammatory effects | |
Adrenal (Medulla) | epinephrine, norepinephrine | stimulate fight-or-flight response; increase blood glucose levels; increase metabolic activities |
Pancreas | insulin | reduces blood glucose levels |
glucagon | increases blood glucose levels | |
Pineal gland | melatonin | regulates some biological rhythms and protects CNS from free radicals |
Testes | androgens | regulate, promote, increase or maintain sperm production; male secondary sexual characteristics |
Ovaries | estrogen | promotes uterine lining growth; female secondary sexual characteristics |
progestins | promote and maintain uterine lining growth |
Learn By Doing 18.8
Which of the following accurately describes the function of the hypothalamus?
- It directly regulates all endocrine secretions.
- It is regulated by the pituitary gland.
- It is responsible for only stimulating the pituitary gland.
- It is a link between the nervous system and the endocrine system.
Which endocrine gland receives hormonal signals secreted by the hypothalamus and transported via the hypophyseal portal vein?
- thymus
- posterior pituitary
- anterior pituitary
- pineal gland
Which hormone(s) secreted by the anterior pituitary control(s) the function of other endocrine glands(s)?
- TSH
- ACTH
- FSH
- All of these are tropic hormones.
What hormone(s) is/are produced by the posterior pituitary? ADH
- ADH
- Oxytocin
- Prolactin
- None of the above
What is the major function of the thyroid hormones?
- regulate magnesium absorption
- increase metabolic rate
- regulate blood pressure
- regulate secretion from the pituitary gland
What hormone(s) is/are produced by the parathyroid glands?
- ATCH
- ADH
- PTH
- LH
The hormones of the adrenal cortex include:
- Steroid hormones
- Androgen hormones
- Epinephrine
- Steroid hormones and Androgen hormones
What hormone is produced by the pineal gland?
- Melatonin
- Serotonin
- Melanin
- Pinealocytes
Which of the following hormones are produced by the pancreas?
- Insulin and glucose
- Insulin and glucagon
- Insulin and calcitonin
- Insulin and glycogen
Which of the following glands is NOT a source of a steroid hormone?
- ovaries
- adrenal gland
- testes
- pancreas
Some glands are stimulated by neurons rather than hormones (tropic) or receptors monitoring an internal stimulus that changes (humoral). Which of the following gland’s secretion is NOT regulated by neural stimuli?
- posterior pituitary
- pancreas
- adrenal
Organs with Secondary Endocrine Functions
There are several organs whose primary functions are non-endocrine, but that also possess endocrine functions. These include the heart, kidneys, intestines, thymus, gonads, and adipose tissue.
The heart possesses endocrine cells in the walls of the atria that are specialized cardiac muscle cells. These cells release the hormone atrial natriuretic peptide (ANP) in response to increased blood volume. High blood volume causes the cells to be stretched, resulting in hormone release. ANP acts on the kidneys to reduce the reabsorption of Na+, causing Na+ and water to be excreted in the urine. ANP also reduces the amounts of renin released by the kidneys and aldosterone released by the adrenal cortex, further preventing the retention of water. In this way, ANP causes a reduction in blood volume and blood pressure and reduces the concentration of Na+ in the blood.
The gastrointestinal tract produces several hormones that aid in digestion. The endocrine cells are located in the mucosa of the GI tract throughout the stomach and small intestine. Some of the hormones produced include gastrin, secretin, and cholecystokinin, which are secreted in the presence of food, and some of which act on other organs such as the pancreas, gallbladder, and liver. They trigger the release of gastric juices, which help to break down and digest food in the GI tract.
While the adrenal glands associated with the kidneys are major endocrine glands, the kidneys themselves also possess endocrine functions. Renin is released in response to decreased blood volume or pressure and is part of the renin-angiotensin-aldosterone system that leads to the release of aldosterone. Aldosterone then causes the retention of Na+ and water, raising blood volume. The kidneys also release calcitriol, which aids in the absorption of Ca2+ and phosphate ions. Erythropoietin (EPO) is a protein hormone that triggers the formation of red blood cells in the bone marrow. EPO is released in response to low oxygen levels. Because red blood cells are oxygen carriers, increased production results in greater oxygen delivery throughout the body. Athletes have used EPO to improve performance, as greater oxygen delivery to muscle cells allows for greater endurance. Because red blood cells increase the viscosity of blood, artificially high levels of EPO can cause severe health risks.
The thymus is found behind the sternum; it is most prominent in infants, becoming smaller in size through adulthood. The thymus produces hormones referred to as thymosins, which contribute to the development of the immune response.
Adipose tissue is a connective tissue found throughout the body. It produces the hormone leptin in response to food intake. Leptin increases the activity of anorexigenic neurons and decreases that of orexigenic neurons, producing a feeling of satiety after eating, thus affecting appetite and reducing the urge for further eating. Leptin is also associated with reproduction. It must be present for GnRH and gonadotropin synthesis to occur. Extremely thin females may enter puberty late; however, if adipose levels increase, more leptin will be produced, improving fertility.
Summary of Organs with Secondary Endocrine Functions
Some organs possess endocrine activity as a secondary function but have another primary function. The heart produces the hormone atrial natriuretic peptide, which functions to reduce blood volume, pressure, and Na+ concentration. The gastrointestinal tract produces various hormones that aid in digestion. The kidneys produce renin, calcitriol, and erythropoietin. Adipose tissue produces leptin, which promotes satiety signals in the brain.
Endocrine System Homeostasis and Integration of Systems
Hormones have a wide range of effects and modulate many different body processes. The key processes that will be examined in this section are hormonal regulation of the excretory system, the reproductive system, metabolism, blood calcium concentrations, growth, and the stress response. We will see how hormones help the body maintain homeostasis by integrating different organ systems.
Hormones have a wide range of effects and modulate many different body processes. The key regulatory processes that will be examined here are those affecting the excretory system, the reproductive system, metabolism, blood calcium concentrations, growth, and the stress response.
Learning Objectives
- Explain how hormones regulate the excretory system
- Discuss the role of hormones in the reproductive system
- Describe how hormones regulate metabolism
- Explain the role of hormones in blood calcium levels
- Explain the role of hormones in growth
- Explain the role of hormones in stress
Hormonal Regulation of the Excretory System
Maintaining a proper water balance in the body is important to avoid dehydration or overhydration (hyponatremia). Osmoreceptors in the hypothalamus monitor water concentration in the body by detecting the concentration of electrolytes in the extracellular fluid. The concentration of electrolytes in the blood rises when there is water loss caused by excessive perspiration, inadequate water intake, or low blood volume due to blood loss. An increase in blood electrolyte levels results in a neuronal signal being sent from the osmoreceptors in hypothalamic nuclei. The pituitary gland has two components: anterior and posterior. The anterior pituitary is composed of glandular cells that secrete protein hormones. The posterior pituitary is an extension of the hypothalamus. It is composed largely of neurons that are continuous with the hypothalamus.
The hypothalamus produces a polypeptide hormone known as antidiuretic hormone (ADH), which is transported to and released from the posterior pituitary gland. The principal action of ADH is to regulate the amount of water excreted by the kidneys. As ADH (which is also known as vasopressin) causes direct water reabsorption from the kidney tubules, salts and wastes are concentrated in what will eventually be excreted as urine. The hypothalamus controls the mechanisms of ADH secretion, either by regulating blood volume or the concentration of water in the blood. Dehydration or physiological stress can cause an increase of osmolarity above 300 mOsm/L, which in turn, raises ADH secretion and water will be retained, causing an increase in blood pressure. ADH travels in the bloodstream to the kidneys. Once at the kidneys, ADH changes the kidneys to become more permeable to water by temporarily inserting water channels, called aquaporins, into the kidney tubules. Water moves out of the kidney tubules through the aquaporins, reducing urine volume. The water is reabsorbed into the capillaries lowering blood osmolarity back toward normal. As blood osmolarity decreases, a negative feedback mechanism reduces osmoreceptor activity in the hypothalamus, and ADH secretion is reduced. ADH release can be reduced by certain substances, including alcohol, which can cause increased urine production and dehydration.
Chronic underproduction of ADH or a mutation in the ADH receptor results in diabetes insipidus. If the posterior pituitary does not release enough ADH, water cannot be retained by the kidneys and is lost as urine. This causes increased thirst, but water taken in is lost again and must be continually consumed. If the condition is not severe, dehydration may not occur, but severe cases can lead to electrolyte imbalances due to dehydration.
Another hormone responsible for maintaining electrolyte concentrations in extracellular fluids is aldosterone, a steroid hormone that is produced by the adrenal cortex. In contrast to ADH, which promotes the reabsorption of water to maintain proper water balance, aldosterone maintains proper water balance by enhancing Na+ reabsorption and K+ secretion from the extracellular fluid of the cells in kidney tubules. Because it is produced in the cortex of the adrenal gland and affects the concentrations of minerals Na+ and K+, aldosterone is referred to as a mineralocorticoid, a corticosteroid that affects ion and water balance. Aldosterone release is stimulated by a decrease in blood sodium levels, blood volume, or blood pressure or by an increase in blood potassium levels. It also prevents the loss of Na+ from sweat, saliva, and gastric juice. The reabsorption of Na+ also results in the osmotic reabsorption of water, which alters blood volume and blood pressure.
Aldosterone production can be stimulated by low blood pressure, which triggers a sequence of chemical releases. When blood pressure drops, the renin-angiotensin-aldosterone system (RAAS) is activated. Cells in the juxtaglomerular apparatus, which regulates the functions of the nephrons of the kidney, detect this and release renin. Renin, an enzyme, circulates in the blood and reacts with a plasma protein produced by the liver called angiotensinogen. When angiotensinogen is cleaved by renin, it produces angiotensin I, which is then converted into angiotensin II in the lungs. Angiotensin II functions as a hormone and then causes the release of the hormone aldosterone by the adrenal cortex, resulting in increased Na+ reabsorption, water retention, and an increase in blood pressure. Angiotensin II in addition to being a potent vasoconstrictor also causes an increase in ADH and increased thirst, both of which help to raise blood pressure.
Learn By Doing 18.9
Which of the following combination of hormones are involved in regulating water homeostasis?
- ACTH, Secretin, Aldosterone
- ADH, Secretin, Aldosterone
- ADH, Gastrin, Angiotension
- ADH, Renin, Aldosterone
Hormonal Regulation of the Reproductive System
Regulation of the reproductive system is a process that requires the action of hormones from the pituitary gland, the adrenal cortex, and the gonads. During puberty in both males and females, the hypothalamus produces gonadotropin-releasing hormone (GnRH), which stimulates the production and release of follicle-stimulating hormone (FSH) and luteinizing hormone (LH) from the anterior pituitary gland. These hormones regulate the gonads (testes in males and ovaries in females) and therefore are called gonadotropins. In both males and females, FSH stimulates gamete production, and LH stimulates the production of hormones by the gonads. An increase in gonad hormone levels inhibits GnRH production through a negative feedback loop.
Regulation of the Male Reproductive System
In males, FSH stimulates the maturation of sperm cells. FSH production is inhibited by the hormone inhibin, which is released by the testes. LH stimulates the production of the sex hormones (androgens) by the interstitial cells of the testes and therefore is also called interstitial cell-stimulating hormone.
The most widely known androgen in males is testosterone. Testosterone promotes the production of sperm and masculine characteristics. The adrenal cortex also produces small amounts of testosterone precursor, although the role of this additional hormone production is not fully understood.
The Dangers of Synthetic Hormones
Some athletes attempt to boost their performance by using artificial hormones that enhance muscle performance. Anabolic steroids, a form of the male sex hormone testosterone, are one of the most widely known performance-enhancing drugs. Steroids are used to help build muscle mass. Other hormones that are used to enhance athletic performance include erythropoietin, which triggers the production of red blood cells, and human growth hormone, which can help in building muscle mass. Most performance-enhancing drugs are illegal for non-medical purposes. National and international governing bodies including the International Olympic Committee, the U.S. Olympic Committee, the National Collegiate Athletic Association, the Major League Baseball, and the National Football League also ban them.
The side effects of synthetic hormones are often significant and non-reversible, and in some cases, fatal. Androgens produce several complications such as liver dysfunctions and liver tumors, prostate gland enlargement, difficulty urinating, premature closure of epiphyseal cartilages, testicular atrophy, infertility, and immune system depression. The physiological strain caused by these substances is often greater than what the body can handle, leading to unpredictable and dangerous effects and linking their use to heart attacks, strokes, and impaired cardiac function.
Regulation of the Female Reproductive System
In females, FSH stimulates the development of egg cells, called ova, which develop in structures called follicles. Follicle cells produce the hormone inhibin, which inhibits FSH production. LH also plays a role in the development of ova, induction of ovulation, and stimulation of estradiol and progesterone production by the ovaries. Estradiol and progesterone are steroid hormones that prepare the body for pregnancy. Estradiol produces secondary sex characteristics in females, while both estradiol and progesterone regulate the menstrual cycle.
In addition to producing FSH and LH, the anterior portion of the pituitary gland also produces the hormone prolactin (PRL) in females. Prolactin stimulates the production of milk by the mammary glands following childbirth. Prolactin levels are regulated by the hypothalamic hormones prolactin-releasing hormone (PRH) and prolactin-inhibiting hormone (PIH), which is now known to be dopamine. PRH stimulates the release of prolactin, and PIH inhibits it.
The posterior pituitary releases the hormone oxytocin, which stimulates uterine contractions during childbirth. The uterine smooth muscles are not very sensitive to oxytocin until late in pregnancy when the number of oxytocin receptors in the uterus peaks. Stretching of tissues in the uterus and cervix stimulates oxytocin release during childbirth. Contractions increase in intensity as blood levels of oxytocin rise via a positive feedback mechanism until the birth is complete.
Oxytocin also stimulates the contraction of myoepithelial cells around the milk-producing mammary glands. As these cells contract, milk is forced from the secretory alveoli into milk ducts and is ejected from the breasts in milk ejection (“let-down”) reflex. Oxytocin release is stimulated by the suckling of an infant, which triggers the synthesis of oxytocin in the hypothalamus and its release into circulation at the posterior pituitary.
Learn By Doing 18.10
FSH and LH from the anterior pituitary regulate the release of hormones from the gonads. Which classification is correct for these two hormones?
- tropic
- neurosecretory
- trophic
- steroid
Testosterone and estrogen from the gonads regulate the production of gametes. Which classification is correct for these two hormones?
- peptide
- tropic
- trophic
- neurosecretory
Two hormones are involved in the combined production and release of milk. Which of them are involved in a positive feedback mechanism?
- oxytocin alone
- both oxytocin and prolactin
- prolactin alone
Which of the following is not a hormone involved in regulating both the male and female reproductive systems?
- FSH
- PRH
- LH
- GnRH
In addition to the testes, what other endocrine gland produces a small amount of testosterone?
- The posterior pituitary
- The adrenal cortex
- The adrenal medulla
- The anterior pituitary
Hormonal Regulation of Metabolism
Blood glucose levels vary widely over the course of a day as periods of food consumption alternate with periods of fasting. Insulin and glucagon are the two hormones primarily responsible for maintaining homeostasis of blood glucose levels. Additional regulation is mediated by the thyroid hormones.
Regulation of Blood Glucose Levels by Insulin and Glucagon
Cells of the body require nutrients in order to function, and these nutrients are obtained through feeding. In order to manage nutrient intake, store excess intake, and utilize reserves when necessary, the body uses hormones to moderate energy stores. Insulin is produced by the beta cells of the pancreas, which are stimulated to release insulin as blood glucose levels rise (for example, after a meal is consumed). Insulin lowers blood glucose levels by enhancing the rate of glucose uptake and utilization by target cells, which use glucose for ATP production. It also stimulates the liver to convert glucose to glycogen, which is then stored by cells for later use. Insulin also increases glucose transport into certain cells, such as muscle cells and the liver. This results from an insulin-mediated increase in the number of glucose transporter proteins in cell membranes, which remove glucose from circulation by facilitated diffusion. As insulin binds to its target cell via insulin receptors and signal transduction, it triggers the cell to incorporate glucose transport proteins into its membrane. This allows glucose to enter the cell, where it can be used as an energy source. However, this does not occur in all cells: some cells, including those in the kidneys and brain, can access glucose without the use of insulin. Insulin also stimulates the conversion of glucose to fat in adipocytes and the synthesis of proteins. These actions mediated by insulin cause blood glucose concentrations to fall called a hypoglycemic “low sugar” effect, which inhibits further insulin release from beta cells through a negative feedback loop.
Impaired insulin function can lead to a condition called diabetes mellitus. This can be caused by low levels of insulin production by the beta cells of the pancreas or by reduced sensitivity of tissue cells to insulin. This prevents glucose from being absorbed by cells, causing high levels of blood glucose or hyperglycemia (high sugar). High blood glucose levels make it difficult for the kidneys to recover all the glucose from nascent urine, resulting in glucose being lost in urine. High glucose levels also result in less water being reabsorbed by the kidneys, causing high amounts of urine to be produced; this may result in dehydration. Over time, high blood glucose levels can cause nerve damage to the eyes and peripheral body tissues, as well as damage to the kidneys and cardiovascular system. Oversecretion of insulin can cause hypoglycemia, called low blood glucose levels. This causes insufficient glucose availability to cells, often leading to muscle weakness, and can sometimes cause unconsciousness or death if left untreated.
When blood glucose levels decline below normal levels, for example between meals or when glucose is utilized rapidly during exercise, the hormone glucagon is released from the alpha cells of the pancreas. Glucagon raises blood glucose levels, eliciting what is called a hyperglycemic effect, by stimulating the breakdown of glycogen to glucose in skeletal muscle cells and liver cells in a process called glycogenolysis. Glucose can then be utilized as energy by muscle cells and released into circulation by the liver cells. Glucagon also stimulates the absorption of amino acids from the blood by the liver, which then converts them to glucose. This type of glucose synthesis is called gluconeogenesis. Glucagon also stimulates adipose cells to release fatty acids into the blood. These actions mediated by glucagon result in an increase in blood glucose levels to normal homeostatic levels. Rising blood glucose levels inhibit further glucagon release by the pancreas via a negative feedback mechanism. In this way, insulin and glucagon work together to maintain homeostatic glucose levels.
Regulation of Blood Glucose Levels by Thyroid Hormones
The basal metabolic rate, which is the number of calories required by the body at rest, is determined by two hormones produced by the thyroid gland: thyroxine, also known as tetraiodothyronine or T4, and triiodothyronine, also known as T3. These hormones affect nearly every cell in the body except for the adult brain, uterus, testes, blood cells, and spleen. They are transported across the plasma membrane of target cells and bind to receptors on the mitochondria resulting in increased ATP production. In the nucleus, T3 and T4 activate genes involved in energy production and glucose oxidation. This results in increased rates of metabolism and body heat production, which is known as the hormone’s calorigenic effect.
T3 and T4 release from the thyroid gland is stimulated by thyroid-stimulating hormone (TSH), which is produced by the anterior pituitary. TSH binding at the receptors of the follicle of the thyroid triggers the production of T3 and T4 from a glycoprotein called thyroglobulin. Thyroglobulin is present in the follicles of the thyroid and is converted into thyroid hormones with the addition of iodine. Iodine is formed from iodide ions that are actively transported into the thyroid follicle from the bloodstream. A peroxidase enzyme then attaches the iodine to the tyrosine amino acid found in thyroglobulin. T3 has three iodine ions attached, while T4 has four iodine ions attached. T3 and T4 are then released into the bloodstream, with T4 being released in much greater amounts than T3. As T3 is more active than T4 and is responsible for most of the effects of thyroid hormones, tissues of the body convert T4 to T3 by the removal of an iodine ion. Most of the released T3 and T4 become attached to transport proteins in the bloodstream and is unable to cross the plasma membrane of cells. These protein-bound molecules are only released when blood levels of the unattached hormone begin to decline. In this way, a week’s worth of reserve hormone is maintained in the blood. Increased T3 and T4 levels in the blood inhibit the release of TSH, which results in lower T3 and T4 release from the thyroid.
The follicular cells of the thyroid require iodides (anions of iodine) in order to synthesize T3 and T4. Iodides obtained from the diet are actively transported into follicle cells resulting in a concentration that is approximately 30 times higher than in blood. The typical diet in North America provides more iodine than required due to the addition of iodide to table salt. Inadequate iodine intake, which occurs in many developing countries, results in an inability to synthesize T3 and T4 hormones. The thyroid gland enlarges in a condition called goiter, which is caused by the overproduction of TSH without the formation of thyroid hormone. Thyroglobulin is contained in a fluid called colloid, and TSH stimulation results in higher levels of colloid accumulation in the thyroid. In the absence of iodine, this is not converted to thyroid hormone, and colloid begins to accumulate more and more in the thyroid gland, leading to goiter.
Disorders can arise from both the underproduction and overproduction of thyroid hormones. Hypothyroidism, underproduction of the thyroid hormones, can cause a low metabolic rate leading to weight gain, sensitivity to colds, and reduced mental activity, among other symptoms. In children, hypothyroidism can cause cretinism, which can lead to mental retardation and growth defects. Hyperthyroidism, the overproduction of thyroid hormones, can lead to an increased metabolic rate and its effects: weight loss, excess heat production, sweating, and an increased heart rate. Graves’ disease is one example of a hyperthyroid condition.
Learn By Doing 18.11
After a meal is consumed, you would expect:
- Blood glucose to go down and glycogen to go up.
- Blood glucose to go down and glycogen to go down.
- Blood glucose to go up and insulin to go down.
- Blood glucose to go up and insulin to go up.
Which of the following is not properly matched?
- Triiodothyronine increases body heat production.
- Glucagon stimulates the conversion of glucose to fatty acids.
- Insulin stimulates glycogen formation.
- Thyroxine increases glucose oxidation.
Which of the following statements is correct:
- Hyperglycemia is associated with too much insulin.
- Hypoglycemia is associated with reduced urine production.
- Hypothyroidism is associated with weight gain, cold-intolerance, and fatigue.
- Hyperthyroidism would be associated with too much iodine.
Hormonal Control of Blood Calcium Levels
Regulation of blood calcium concentrations is important for the generation of muscle contractions and nerve impulses, which are electrically stimulated. If calcium levels get too high, membrane permeability to sodium decreases, and membranes become less responsive. If calcium levels get too low, membrane permeability to sodium increases, and convulsions or muscle spasms can result.
Blood calcium levels are regulated by parathyroid hormone (PTH), which is produced by the parathyroid glands. PTH is released in response to low blood Ca2+ levels. PTH increases Ca2+ levels by targeting the skeleton, the kidneys, and the intestine. In the skeleton, PTH stimulates osteoclasts, which causes bone to be reabsorbed, releasing Ca2+ from bone into the blood. PTH also inhibits osteoblasts, reducing Ca2+ deposition in bone. In the intestines, PTH increases dietary Ca2+ absorption, and in the kidneys, PTH stimulates the reabsorption of the Ca2+. While PTH acts directly on the kidneys to increase Ca2+ reabsorption, its effects on the intestine are indirect. PTH triggers the formation of calcitriol, an active form of vitamin D, which acts on the intestines to increase the absorption of dietary calcium. PTH release is inhibited by rising blood calcium levels.
Hyperparathyroidism results from an overproduction of parathyroid hormone. This results in excessive calcium being removed from bones and introduced into blood circulation, producing structural weakness of the bones, which can lead to deformation and fractures, plus nervous system impairment due to high blood calcium levels. Hypoparathyroidism, the underproduction of PTH, results in extremely low levels of blood calcium, which causes impaired muscle function and may result in tetany (severe sustained muscle contraction).
The hormone calcitonin, which is produced by the parafollicular or C cells of the thyroid, has the opposite effect on blood calcium levels as does PTH. Calcitonin decreases blood calcium levels by inhibiting osteoclasts, stimulating osteoblasts, and stimulating calcium excretion by the kidneys. This results in calcium being added to the bones to promote structural integrity. Calcitonin is most important in children (when it stimulates bone growth), during pregnancy (when it reduces maternal bone loss), and during prolonged starvation (because it reduces bone mass loss). In healthy nonpregnant, un-starved adults, the role of calcitonin is unclear.
Learn By Doing 18.12
Which of the following would not be stimulated by calcitonin?
- decreased intestinal absorption
- increased calcium in urine
- osteoporosis
- decreased parathyroid hormone
Which of the following is not a target of parathyroid hormone?
- Kidneys
- Skeleton
- Intestine
- Skin
Hormonal Regulation of Growth
Hormonal regulation is required for the growth and replication of most cells in the body. Growth hormone (GH), produced by the anterior portion of the pituitary gland, accelerates the rate of protein synthesis, particularly in skeletal muscle and bones. Growth hormone has direct and indirect mechanisms of action. The first direct action of GH is the stimulation of triglyceride breakdown (lipolysis) and release into the blood by adipocytes. This results in a switch by most tissues from utilizing glucose as an energy source to utilizing fatty acids. This process is called a glucose-sparing effect. In another direct mechanism, GH stimulates glycogen breakdown in the liver; the glycogen is then released into the blood as glucose. Blood glucose levels increase as most tissues are utilizing fatty acids instead of glucose for their energy needs. The GH-mediated increase in blood glucose levels is called a diabetogenic effect because it is similar to the high blood glucose levels seen in diabetes mellitus.
The indirect mechanism of GH action is mediated by insulin-like growth factors (IGFs) or somatomedins, which are a family of growth-promoting proteins produced by the liver that stimulate tissue growth. IGFs stimulate the uptake of amino acids from the blood, allowing the formation of new proteins, particularly in skeletal muscle cells, cartilage cells, and other target cells. This is especially important after a meal when glucose and amino acid concentration levels are high in the blood. Two hormones produced by the hypothalamus regulate GH levels. GH release is stimulated by growth hormone-releasing hormone (GHRH) and is inhibited by growth hormone-inhibiting hormone (GHIH), also called somatostatin.
A balanced production of growth hormone is critical for proper development. The underproduction of GH in adults does not appear to cause any abnormalities, but in children, it can result in pituitary dwarfism, in which growth is reduced. Pituitary dwarfism is characterized by symmetric body formation. In some cases, individuals are under 30 inches in height. Oversecretion of growth hormone can lead to gigantism in children, causing excessive growth. In some documented cases, individuals can reach heights of over eight feet. In adults, excessive GH can lead to acromegaly, a condition in which there is the enlargement of bones in the face, hands, and feet that are still capable of growth.
Learn By Doing 18.13
What is the effect of an overproduction of growth hormone in children and in adults?
- In children it can cause excessive proportional growth; in adults, it primarily affects the bones of the face, hands, and feet.
- It has the same effect of causing excessive growth of all bones in both children and adults. In children it can cause pituitary dwarfism; in adults, it primarily affects the bones of the face, hands, and feet.
- In children it primarily affects the bones of the face, hands, and feet; in adults, it can cause excessive proportional growth.
Hormonal Regulation of Stress
When a threat or danger is perceived, the body responds by releasing hormones that will ready it for the “fight-or-flight” response. The effects of this response are familiar to anyone who has been in a stressful situation: increased heart rate, dry mouth, and hair standing up.
Fight-or-Flight Response
Interactions of the endocrine hormones have evolved to ensure the body’s internal environment remains stable. Stressors are stimuli that disrupt homeostasis. The sympathetic division of the vertebrate autonomic nervous system has evolved the fight-or-flight response to counter stress-induced disruptions of homeostasis. In the initial alarm phase, the sympathetic nervous system stimulates an increase in energy levels through increased blood glucose levels. This prepares the body for physical activity that may be required to respond to stress: to either fight for survival or to flee from danger.
However, some stresses, such as illness or injury, can last for a long time. Glycogen reserves, which provide energy in the short-term response to stress, are exhausted after several hours and cannot meet long-term energy needs. If glycogen reserves were the only energy source available, neural functioning could not be maintained once the reserves became depleted due to the nervous system’s high requirement for glucose. In this situation, the body has evolved a response to counter long-term stress through the actions of glucocorticoids, which ensure that long-term energy requirements can be met. The glucocorticoids mobilize lipid and protein reserves, stimulate gluconeogenesis, conserve glucose for use by neural tissue, and stimulate the conservation of salts and water. The mechanisms to maintain homeostasis that are described here are those observed in the human body. However, the fight-or-flight response exists in some form in all vertebrates.
The sympathetic nervous system regulates the stress response via the hypothalamus. Stressful stimuli cause the hypothalamus to signal the adrenal medulla (which mediates short-term stress responses) via nerve impulses, and the adrenal cortex, which mediates long-term stress responses, via the hormone adrenocorticotropic hormone (ACTH), which is produced by the anterior pituitary.
Short-Term Stress Response
When presented with a stressful situation, the body responds by calling for the release of hormones that provide a burst of energy. The hormones epinephrine (also known as adrenaline) and norepinephrine (also known as noradrenaline) are released by the adrenal medulla. How do these hormones provide a burst of energy? Epinephrine and norepinephrine increase blood glucose levels by stimulating the liver and skeletal muscles to break down glycogen and by stimulating glucose release by liver cells. Additionally, these hormones increase oxygen availability to cells by increasing the heart rate and dilating the bronchioles. The hormones also prioritize body function by increasing blood supply to essential organs such as the heart, brain, and skeletal muscles, while restricting blood flow to organs not in immediate need, such as the skin, digestive system, and kidneys. Epinephrine and norepinephrine are collectively called catecholamines.
Long-Term Stress Response
Long-term stress response differs from the short-term stress response. The body cannot sustain the bursts of energy mediated by epinephrine and norepinephrine for long times. Instead, other hormones come into play. In a long-term stress response, the hypothalamus triggers the release of ACTH from the anterior pituitary gland. ACTH stimulates the adrenal cortex to release steroid hormones called corticosteroids. Corticosteroids turn on the transcription of certain genes in the nuclei of target cells. They change enzyme concentrations in the cytoplasm and affect cellular metabolism. There are two main corticosteroids: glucocorticoids such as cortisol, and mineralocorticoids such as aldosterone. These hormones target the breakdown of fat into fatty acids in the adipose tissue. The fatty acids are released into the bloodstream for other tissues to use for ATP production. The glucocorticoids primarily affect glucose metabolism by stimulating glucose synthesis. Glucocorticoids also have anti-inflammatory properties through inhibition of the immune system. For example, cortisone is used as an anti-inflammatory medication; however, it cannot be used long-term as it increases susceptibility to disease due to its immune-suppressing effects.
Mineralocorticoids function to regulate ion and water balance of the body. The hormone aldosterone stimulates the reabsorption of water and sodium ions in the kidney, which results in increased blood pressure and volume.
Hypersecretion of glucocorticoids can cause a condition known as Cushing’s disease, characterized by a shifting of fat storage areas of the body. This can cause the accumulation of adipose tissue in the face and neck and excessive glucose in the blood. Hyposecretion of the corticosteroids can cause Addison’s disease, which may result in bronzing of the skin, hypoglycemia, and low electrolyte levels in the blood.
Learn By Doing 18.14
Which of the following hormones is involved in short-term stress?
- corticosteroids
- epinephrine
- cortisol
- adrenocorticotropic hormone
Which hormone is not responsible for the long-term stress response?
- Cortisol
- ACTH
- Adrenaline
- Aldosterone
Summary
Water levels in the body are controlled by antidiuretic hormone (ADH), which is produced in the hypothalamus and triggers the reabsorption of water by the kidneys. The underproduction of ADH can cause diabetes insipidus. Aldosterone, a hormone produced by the adrenal cortex of the kidneys, enhances Na+ reabsorption from the extracellular fluids and subsequent water reabsorption by diffusion. The renin-angiotensin-aldosterone system is one way that aldosterone release is controlled.
The reproductive system is controlled by the gonadotropins follicle-stimulating hormone (FSH) and luteinizing hormone (LH), which are produced by the pituitary gland. Gonadotropin release is controlled by the hypothalamic hormone gonadotropin-releasing hormone (GnRH). FSH stimulates the maturation of sperm cells in males and is inhibited by the hormone inhibin, while LH stimulates the production of the androgen testosterone. FSH stimulates egg maturation in females, while LH stimulates the production of estrogens and progesterone. Estrogens are a group of steroid hormones produced by the ovaries that trigger the development of secondary sex characteristics in females as well as control the maturation of the ova. In females, the pituitary also produces prolactin, which stimulates milk production after childbirth, and oxytocin, which stimulates uterine contraction during childbirth and milk let-down during suckling.
Insulin is produced by the pancreas in response to rising blood glucose levels and allows cells to utilize blood glucose and store excess glucose for later use. Diabetes mellitus is caused by reduced insulin activity and causes high blood glucose levels called hyperglycemia. Glucagon is released by the pancreas in response to low blood glucose. It stimulates the breakdown of glycogen into glucose, which the body can use. The body’s basal metabolic rate is controlled by the thyroid hormones thyroxine (T4) and triiodothyronine (T3). The anterior pituitary produces thyroid stimulating hormone (TSH), which controls the release of T3 and T4 from the thyroid gland. Iodine is necessary for the production of thyroid hormone, and the lack of iodine can lead to a condition called goiter.
*
“Learn By Doing” and “Did I Get This?” Feedback
Learn By Doing 18.1
Decide if the nervous or endocrine system is responsible for each of the following:
- Chemicals transported in the blood
This is true of hormones but not true of neurotransmitters, although both neurons and endocrine gland cells, such as epinephrine from the nervous system and adrenal gland can release some chemicals. Hormones are transported in the blood but only affect those cells with a specific receptor for that hormone. - Quick initiation and conclusion of regulatory effect in the body
Neuron stimulation occurs within milliseconds and is quickly degraded unless more neurotransmitter is released through continued stimulation. Once released, hormones must travel to the receptors, and they often circulate in the blood for days with some concentration level. - Slow initiation but the long-term regulatory effect on the body
Once released, the hormones must travel to the receptors, and they often circulate in the blood for days with some concentration level. In contrast, each action potential is quick, and the release of neurotransmitters is only continued with continued stimulation of the neuron. - Directly regulates the metabolism of tissues and cells
Hormones affect the metabolism of their target cells and tissues, while neurons do not.
Learn By Doing 18.2
If you had to summarize the function of the endocrine system in one word, what would it be?
- Communication
Incorrect. Communication is the method that the endocrine system uses to maintain homeostasis. - Homeostasis
Correct. The endocrine system’s main function is to maintain homeostasis. However, growth, development, and communication are also important endocrine system functions. - Development
Incorrect. The endocrine system does influence development, but the system’s control of development is tied to homeostasis. - Growth
Incorrect. The endocrine system does influence growth, but the system’s control of growth is tied to homeostasis.
Which of the following is not a function of the endocrine system?
- Produce quick effects through electrochemical mechanisms.
Correct. This is a function of the nervous system, not the endocrine system, which uses hormones for a slower but longer-duration effect on sometimes distant body tissues and cells. - Release chemicals into the bloodstream for distribution throughout the body.
Incorrect. This is indeed a function of the endocrine system; those chemicals are called hormones. - Contribute to homeostatic feedback loops.
Incorrect. This is indeed a function of the endocrine system, although the nervous system also functions in this way. - Alter the metabolic activities of many different target tissues and organs.
Incorrect. This is indeed a function of the endocrine system; there are different mechanisms to affect target cell metabolism.
Endocrine glands ________.
- secrete hormones that travel through a duct to the target organs
- release neurotransmitters into the synaptic cleft
- secrete chemical messengers that travel in the bloodstream
- include sebaceous glands and sweat glands
Learn By Doing 18.3
Which of the following accurately describes hormones?
- Circulate indefinitely in the body
Incorrect. Hormones will degrade or eventually will be eliminated. - Are chemical messengers
Correct. Hormones are chemical messengers. - Are neural messengers
Incorrect. Neural messages are carried through electrical impulses. - Elicit a body-wide response from all cell types
Incorrect. Hormones can only elicit responses from target cells.
A cell that has a receptor for a specific hormone is called a:
- Target cell
Correct. Cells that have the receptor for a specific hormone is the target cell for that hormone. - Specialized cell
Incorrect. Although these cells are considered to be specialized when discussing the specific receptors, it is a target cell. - Differentiated cell
Incorrect. These cells have differentiated, but when discussing the specific receptors, it is a target cell. - Endocrine cell
Incorrect. Endocrine cells produce hormones, although some endocrine cells can have receptors for hormones.
What would happen to a body cell that doesn’t have a specific receptor for a specific hormone that is released and circulating in the blood?
- The cell would decrease its physiological response to that hormone.
Incorrect. Without a receptor, there would be no mechanism to influence the cell’s metabolism. - The cell would not respond to the hormone.
Correct. A cell will only respond if it has a receptor for a specific hormone, regardless of the amount circulating and coming into contact with that cell. - The cell would increase its physiological response to that hormone
Incorrect. Without a receptor, there would be no mechanism to influence the cell’s metabolism.
What would happen to a body cell if another chemical, such as a drug, completely blocked a specific hormone receptor?
- The cell would die.
Incorrect. - The cell would not respond to the hormone.
Correct. If the receptors were blocked completely, it is as if the cell did not have any receptors for that hormone. - The cell would increase its physiological response to that hormone.
Incorrect. Some drugs can enhance a hormone effect but by activating the receptor.
Learn By Doing 18.4
Which of the following is correctly matched?
- Steroid hormones bind to a nuclear receptor. Thyroid hormones bind to a cytoplasmic receptor.
Incorrect. Steroid hormones bind to a receptor in the cytoplasm, and the resulting complex stimulates the DNA; thyroid hormones bind to intracellular but not cytoplasmic receptors. - Steroid hormones bind to a cytoplasmic receptor. Thyroid hormones bind to a nuclear receptor.
Correct. Steroid hormones bind to a cytoplasmic receptor forming a complex that then stimulates transcription and thyroid hormones bind to nuclear receptors that inhibit transcription until the hormone binds. - Steroid hormones bind to an intracellular receptor. Thyroid hormones bind to a plasma membrane receptor.
Incorrect. Steroid hormones do bind to an intracellular receptor in the cytoplasm, but so do thyroid hormones. - Steroid hormones bind to a plasma membrane receptor. Thyroid hormones bind to an intracellular receptor.
Incorrect. Both of these hormones bind to some intracellular receptors.
Peptide hormones must bind to a plasma membrane hormone receptor because:
- They are too big.
Incorrect. Some peptide hormones may be large, but it is not their size that keeps them from passing through the membrane. - They are nonpolar.
Incorrect. They are polar meaning they will not be able to pass through the nonpolar portion of the plasma membrane. - They are polar.
Correct. They are polar meaning they will not be able to pass through the nonpolar portion of the plasma membrane. - They are small.
Incorrect. Some peptide hormones may be small, but it is not their size that keeps them from passing through the membrane.
A newly developed pesticide has been observed to bind to an intracellular hormone receptor. If ingested, residue from this pesticide could disrupt the effects of ________.
- melatonin
- estrogen (Estrogen is a lipid.)
- growth hormone
- insulin
A small molecule binds to a G protein, preventing its activation. What direct effect will this have on signaling that involves cAMP?
- The hormone will not be able to bind to the hormone receptor.
- Adenyl cyclase will not be activated.
- Excessive quantities of cAMP will be produced.
- The phosphorylation cascade will be initiated.
Why is it important to know the location of a hormone receptor?
- It is important because it provides information about how the hormone will affect the cell.
Correct. By knowing if the receptor is membrane-bound or internal certain predictions about the mechanism of its action can be made. - It is important because it tells exactly what hormone it will bind.
Incorrect. The structure of the receptor will determine what hormone it binds. - It is important because it tells what type of hormone will be produced.
Incorrect. The receptor is located on the target cell, not the hormone-producing cell; a receptor is what a hormone binds to. - It is not important because all receptors will work the same way.
Incorrect. Knowing where the receptor is located can allow predictions about its mechanism to be made.
Learn By Doing 18.5
Using this diagram to illustrate the childbirth example, which letter on this positive feedback loop image would represent the release of the hormone oxytocin?
- A Incorrect. The initial mechanical stimulus from the baby would this part.
- B Incorrect. This would be a stretch of the cervix, whether an initial event or continued because of the positive feedback effect.
- C Incorrect. The receptors use nerve action potentials to communicate with the control center.
- D Correct. The hormone is released by the control center and travels in the blood to the cervix effector to stimulate more smooth muscle contraction.
- E Incorrect. This would be the continued stretch of the cervix because of the positive feedback effect.
For oxytocin, what type of receptor would be involved in this positive feedback loop?
- chemoreceptor
Incorrect. The receptor is not responding to the hormone chemical; instead, the stretching is stimulating the release of a chemical that causes a change in the effector. - thermoreceptor
Incorrect. Thermoreceptors are not involved in this feedback loop although they are involved in body temperature homeostasis through a different loop. - mechanoreceptor
Correct. The mechanoreceptors detect the push of the fetus against the cervix and uterine walls as labor is initiated. - photoreceptor
Incorrect. The only photoreceptors in humans are located in the retina of the eye.
For oxytocin, what would be the control center?
- cervix
Incorrect. This is the location of the receptor. - mammary gland
Incorrect. This response to oxytocin for lactation is another target effector for a different positive feedback loop. - pancreas
Incorrect. The pancreas releases both exocrine secretions and endocrine hormones, but not oxytocin. - pituitary
Correct. As you will learn later, it is specifically the combined hypothalamus and posterior pituitary, also known as the neurohypophysis.
The cervical and uterine tissue becomes more sensitive to oxytocin during late pregnancy in response to increased levels of estrogen in the mother’s blood. What might be changing to make this tissue more sensitive to oxytocin and enhance this positive feedback?
Our Answer: The steroid estrogen hormone is stimulating the transcription of protein receptors for oxytocin that are inserted into the smooth muscle cell membrane. Oxytocin binds to its receptor to stimulate stronger and longer contractions for birth.
A positive feedback loop is unstable and would result in a runaway situation if something did not terminate the continued increased stimulus. In the example of childbirth, why does the feedback loop stop eventually?
Our Answer: After the baby is born, the stretch receptors are no longer stimulated, and the release of oxytocin decreases.
The contractions will continue for minutes to an hour after the baby is born. This helps expel the placenta, also known as afterbirth. Why would the contractions continue for this time period even though the baby has been born?
Our Answer: Hormones produced before the end of stretch receptors being stimulated are still circulating in the blood and will continue to stimulate the uterine smooth muscle with receptors for oxytocin until the hormone is bound or degraded slowly. Unlike the nervous system which initiates and ends stimulation quickly, the endocrine system is not only slower to initiate but also slower to end its effect.
Learn By Doing 18.6
This diagram illustrates the negative feedback mechanism involving three hormones.
Which letter on this negative feedback loop image would represent the release of the hormone that is the “opposite change” restoring homeostasis?
- A: Incorrect. The thyroid-releasing hormone has an effect on the anterior pituitary but is not itself the hormone being monitored and controlled homeostatically.
- B: Incorrect. The thyroid stimulating hormone has an effect on the thyroid gland but is not itself the hormone being monitored and controlled homeostatically.
- C: Correct. The two thyroid hormones (T3 and T4) are increasing as a direct opposite change from the stimulus which was a decrease in their plasma concentration.
- D: Incorrect. The resulting increased level of the thyroid hormones back into the homeostatic range provides negative feedback to stop the loop to prevent too much from being produced.
- E: Incorrect. The indirect effect would stimulate the additional production of thyroid hormone even if the thyroid hormone level was within the homeostatic range, facilitating a response temporarily not controlled by the negative feedback loop.
In this negative feedback loop, there are two “control centers” receiving feedback from the change initiated by the effector. One is neural, and one is endocrine. A similar control relationship between these two exists for several tropic hormones that control other endocrine glands. Based on this, which endocrine gland is sometimes called the “master gland”?
- anterior pituitary gland
Correct. This endocrine gland produces a variety of hormones under the control of the hypothalamus, many of which then control other endocrine glands. - posterior pituitary gland
Incorrect. This part of the pituitary only stores and releases hormones produced in the hypothalamus, such as oxytocin involved in a positive feedback loop. - hypothalamus
Incorrect. This is the “master” of the “master gland” by releasing both stimulating and inhibiting hormones to control the gland’s production of several tropic hormones. - thyroid gland
Incorrect. This is the final “effector” increasing the production of the hormone in response to its detected decrease in the blood plasma.
Look at the letter “E” on the feedback diagram. What would be the advantage of input from other parts of the brain continuing to stimulate the hypothalamus, and thus more thyroid hormone production even if the blood level has returned within the normal homeostatic range?
Our Answer: Temporarily increased levels of thyroid-releasing and stimulating hormone would result in elevated thyroid hormone levels above the homeostatic range. This would allow the body to generate metabolic heat in an emergency situation, improving cold tolerance along with other homeostatic body temperature regulating mechanisms. This response is most noticeable in young children.
Elevated levels of cortisol, also known as the “stress hormone”, can inhibit the production of thyroid-stimulating hormone from the anterior pituitary. What would be the effect on the production of thyroid hormone by the thyroid gland?
- increased production of thyroid hormone
Incorrect. This would result from an increase in TSH, not its inhibition. - decreased production of thyroid hormone
Correct. Even as thyroid plasma levels fell, the hypothalamus would not be able to stimulate the thyroid without the production of the tropic TSH hormone by the anterior pituitary. - stable production of thyroid hormone
Incorrect. The absence of TSH would definitely affect the thyroid gland’s hormone production.
The autoimmune syndrome Grave’s disease results in antibodies in the blood that bind to thyroid-stimulating hormone receptors, mimicking their metabolic effect on the target cell. What would be the effect on the production of thyroid hormone by the thyroid gland?
- increased production of thyroid hormone
Incorrect. The receptors would be stimulated by not only the hormone but also this antibody, negating the negative feedback control to maintain homeostasis. - decreased production of thyroid hormone
Incorrect. The antibody is chemically behaving as TSH and would definitely affect the thyroid gland’s hormone production. - stable production of thyroid hormone
Correct. Even though the negative feedback reduces the production of releasing and stimulating hormones, the antibodies would continue to stimulate thyroid hormone production.
A different autoimmune disease called Hashimoto’s thyroiditis results in the destruction of thyroid cells by lymphocytes. What would be the effect on the production of thyroid hormone by the thyroid gland?
- increased production of thyroid hormone
Incorrect. Destruction of the endocrine cells would definitely affect the thyroid gland’s ability to produce hormones. - decreased production of thyroid hormone
Correct. The reduction in hormone-producing cells would reduce the gland’s ability to produce sufficient hormone even with sufficient TRH and TSH production. - stable production of thyroid hormone
Incorrect. The remaining cells that the autoimmune response has not destroyed would not be able to produce more hormone.
What would be the advantage of having the negative feedback from the increased thyroid hormones to both the hypothalamus and the anterior pituitary?
Our Answer: By providing feedback to “stop” the continued release of both hypothalamus-releasing hormone and anterior pituitary stimulating hormone, the thyroid will more quickly stop releasing more hormone. Without this, it would take much longer for the hypothalamus to stop stimulating the anterior pituitary and then the anterior pituitary to stop stimulating the thyroid, and the level of circulating thyroid hormone would fluctuate more and potentially increase above the homeostatic range. This situation, however, also makes it hard to artificially mimic the homeostatic maintenance of blood thyroid hormone levels with drug replacements. It often takes weeks or months of adjusting drug doses to treat hypothyroidism or hyperthyroidism with drugs.
Did I Get This? 18.1
Which method of hormone production is going to help return the body to homeostasis?
- Positive Feedback
Incorrect. Positive feedback is going to produce hormones that are going to move the body away from homeostasis until a signal to stop is given. - Negative Feedback
Correct. Negative feedback is the method our body uses to maintain homeostasis by causing a release of hormones that returns the body to its normal state.
Homeostatic mechanisms stimulated by changes in body fluid composition (humoral) often involve more than one hormone in a negative feedback loop. Why would this be typical? For example, insulin decreases blood glucose while glucagon increases blood glucose; parathyroid hormone increases blood calcium levels while calcitonin from the thyroid decreases blood calcium in children.
Our Answer: Stimulating opposite responses to maintain body fluid homeostasis will need to involve different target cells and metabolic responses. Because each hormone has a specific receptor and specific effect on its target cells, it will take different hormones to maintain this humoral component within the homeostatic range. As it increases, it would stimulate the release of one hormone but not the other; as it decreases, it would stimulate the release of the other hormone.
Learn By Doing 18.7
Hormones that control the release of another hormone are called?
- Trophic
Incorrect. Trophic hormones stimulate growth and development. - Tropic
Correct. Tropic means to turn toward and regulate other hormones. - Humor
Incorrect. Humor is a term for bodily fluid. - Triggers
Incorrect. The technical term for these hormones is not “trigger” although their function is to trigger the release of another hormone.
Learn By Doing 18.8
Which of the following accurately describes the function of the hypothalamus?
- It directly regulates all endocrine secretions.
- It is regulated by the pituitary gland.
- It is responsible for only stimulating the pituitary gland.
- It is a link between the nervous system and the endocrine system.
Which endocrine gland receives hormonal signals secreted by the hypothalamus and transported via the hypophyseal portal vein?
- thymus
- posterior pituitary
- anterior pituitary
- pineal gland
Which hormone(s) secreted by the anterior pituitary control(s) the function of other endocrine gland(s)?
- TSH
- ACTH
- FSH
- All of these are tropic hormones.
What hormone(s) are produced by the posterior pituitary?
- ADH
- Oxytocin
- Prolactin
- None of the above
What is the major function of the thyroid hormones?
- regulate magnesium absorption
- increase metabolic rate
- regulate blood pressure
- regulate secretion from the pituitary gland
What hormone(s) are produced by the parathyroid glands?
- ATCH
- ADH
- PTH
- LH
The hormones of the adrenal cortex include:
- Steroid hormones
- Androgen hormones
- Epinephrine
- Steroid hormones and Androgen hormones
What hormone is produced by the pineal gland?
- Melatonin
- Serotonin
- Melanin
- Pinealocytes
Which of the following hormones does the pancreas produce?
- Insulin and glucose
- Insulin and glucagon
- Insulin and calcitonin
- Insulin and glycogen
Which of the following glands is NOT a source of a steroid hormone?
- ovaries
- adrenal gland
- testes
- pancreas
Some glands are stimulated by neurons rather than hormones (tropic) or receptors monitoring an internal stimulus that changes (humoral). Which of the following gland’s secretion is NOT regulated by neural stimuli?
- posterior pituitary
- pancreas
- adrenal
Learn By Doing 18.9
Which of the following combination of hormones are involved in regulating water homeostasis?
- ACTH, Secretin, Aldosterone
- ADH, Secretin, Aldosterone
- ADH, Gastrin, Angiotension
- ADH, Renin, Aldosterone
Learn By Doing 18.10
FSH and LH from the anterior pituitary regulate the release of hormones from the gonads. Which classification is correct for these two hormones?
- tropic(These stimulate the release of testosterone from the testes and estrogen and progesterone from the ovary.)
- neurosecretory
- trophic
- steroid
Testosterone and estrogen from the gonads regulate the production of gametes. Which classification is correct for these two hormones?
- peptide
- tropic
- trophic(In addition to gamete production, testosterone affects the growth of muscle and other tissues while estrogen influences the distribution of adipose and development of the uterus lining in preparation for implantation of a fertilized ovum.)
- neurosecretory
Two hormones are involved in the combined production and release of milk. Which of them are involved in a positive feedback mechanism?
- oxytocin alone (As with the birth process, plasma levels of oxytocin stimulate more oxytocin to be released; in this case, it stimulates stronger contractions for the release of more milk as the baby grows.)
- both oxytocin and prolactin
- prolactin alone
Which of the following is not a hormone involved in regulating both the male and female reproductive systems?
- FSH
- PRH (PRH stands for prolactin-releasing hormone and it is active in females only; although males produce prolactin, it is thought to be stress-regulated and its function is not well understood.)
- LH
- GnRH
In addition to the testes, what other endocrine gland produces a small amount of testosterone?
- The posterior pituitary
- The adrenal cortex
- The adrenal medulla
- The anterior pituitary
Learn By Doing 18.11
After a meal is consumed, you would expect:
- Blood glucose to go down and glycogen to go up.
- Blood glucose to go down and glycogen to go down.
- Blood glucose to go up and insulin to go down.
- Blood glucose to go up and insulin to go up. (After a meal the glucose from the meal will enter the bloodstream and the blood glucose level will go up. And insulin will be produced to lower the blood glucose level.)
Which of the following is not properly matched?
- Triiodothyronine increases body heat production.
- Glucagon stimulates the conversion of glucose to fatty acids. (Glucagon stimulates the breakdown of fatty acids to raise the glucose level of the blood.)
- Insulin stimulates glycogen formation.
- Thyroxine increases glucose oxidation.
Which of the following statements is correct:
- Hyperglycemia is associated with too much insulin.
- Hypoglycemia is associated with reduced urine production.
- Hypothyroidism is associated with weight gain, cold intolerance, and fatigue. (Thyroid hormones stimulate proper metabolic rates, producing enough energy to maintain body temperature and body functions.)
- Hyperthyroidism would be associated with too much iodine.
Learn By Doing 18.12
Which of the following would not be stimulated by calcitonin?
- decreased intestinal absorption
- increased calcium in urine
- osteoporosis(Calcitonin would inhibit osteoclast resorption from bone and is being investigated for its possible use in treating osteoporosis and other bone diseases.)
- decreased parathyroid hormone
Which of the following is not a target of parathyroid hormone?
- Kidneys
- Skeleton
- Intestine
- Skin (The precursor for vitamin D production is produced by the skin, but PTH triggers the active form of this in the intestines.)
Learn By Doing 18.13
What is the effect of an overproduction of growth hormone in children and in adults?
- In children it can cause excessive proportional growth; in adults, it primarily affects the bones of the face, hands, and feet.
- It has the same effect of causing excessive growth of all bones in both children and adults. In children it can cause pituitary dwarfism; in adults, it primarily affects the bones of the face, hands, and feet.
- In children it primarily affects the bones of the face, hands, and feet; in adults, it can cause excessive proportional growth.
Learn By Doing 18.14
Which of the following hormones is involved in short-term stress?
- corticosteroids
- epinephrine
- cortisol
- adrenocorticotropic hormone
Which hormone is not responsible for the long-term stress response?
- Cortisol
- ACTH
- Adrenaline
- Aldosterone